Flash Physics is our daily pick of the latest need-to-know developments from the global physics community selected by Physics World‘s team of editors and reporters

Spotty lizards are cellular automatons
A lizard can change its spots, and now scientists have shown that the process is a living version of a popular computing algorithm. The ocellated lizard begins life with brown skin that is peppered with white spots. As the creature ages, the skin pattern transforms to a labyrinthine pattern in which each individual scale is either green or black. Now, a team of scientists in Switzerland and Russia has shown that this transformation is governed by a process that they call a “living cellular automaton”. In the field of computing, a cellular automaton consists of connected cells whose individual behaviour depends on the states of neighbouring cells. Computational cellular automatons have been used in a wide range of applications from simulating biological systems to creating computer games. In this latest work, Liana Manukyan of the University of Geneva and colleagues used a high-resolution system of robotic cameras to image the skin of three male lizards as they grew to adulthood. This allowed them to watch about 1500 scales on the back of each lizard change colour over a period of four years. Unlike many other animals, in which colour patterns are determined by interactions within individual cells, the results suggest that the colour of an individual cell (lizard scale) is determined by that of its neighbours. The team says that this is the first time that a cellular automaton has been seen in a living organism. Further studies into the chemical and biological processes involved in the skin transformation could provide important insights into why patterns emerge in living systems. The study is described in Nature.
Graphene keeps a lid on liquid analysis
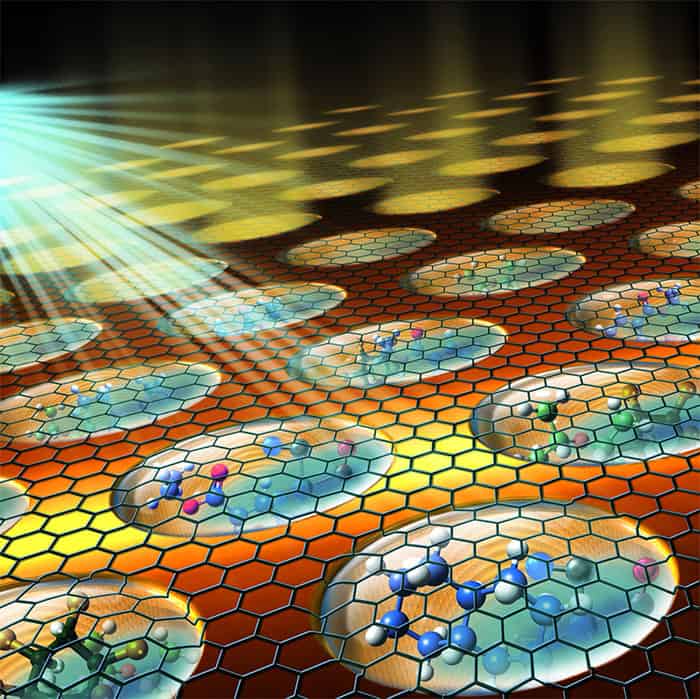
Graphene lids allow liquids to be analysed with a technique usually limited to solid samples. Andrei Kolmakov, from the National Institute of Standards and Technology (NIST) in the US and colleagues have developed a carbon-capped liquid sample array to extend the capability of photoemission electron microscopy (PEEM). The analysis tool involves bombarding a sample with ultraviolet light or X-rays. The photons transfer energy to electrons within the sample, allowing them to escape the material if they are near to the surface. The energy of an emitted electron is specific to the atom it came from, and therefore by using a series of electric lenses and detection systems, PEEM can create an image of the sample’s chemical make-up. Although a popular and powerful tool, PEEM is usually restricted to solid surfaces as liquid samples evaporate and create sparks under the required high vacuum. Kolmakov and team used graphene – an atomically thin sheet of carbon – to seal liquid or gas samples within a multi-channel array. Once the system is under vacuum, the samples are constrained to their channels and remain at atmospheric pressure, but photons and electrons can pass through the graphene nearly completely unimpeded. The simple solution, described in Nano Letters, allows researchers to analyse liquid interfaces and the surface of nanometre-scale objects immersed within liquid, potentially leading to the advancement of batteries and chemical catalysts.
Electron pulses outrun atoms
Electron pulses so short that atoms have no chance to move as the pulses pass through them have been produced by scientists working on the Pegasus radiation facility at the University of California, Los Angeles. The pulses are less than 10 fs in duration and could be used to do time-resolved electron microscopy studies such as following the motions of individual atoms in materials undergoing structural reorganization. Jared Maxson and colleagues created electron pulses of less than 10 fs duration using an electron source similar to those used to deliver electron bunches to synchrotron storage rings. The process begins with firing a 100 fs laser pulse at a cathode, which ejects a pulse of electrons. This relatively long pulse is then sent down a linear accelerator, where it is compressed in time to 10 fs. The final energy of the pulses – which each contain about 500,000 electrons – is several MeV, which is higher than the 50–300 keV used in conventional electron microscopes. While using higher-energy pulses does introduce several challenges, it also offers advantages that arise from relativistic effects in the higher-energy pulses. The research is described in Physical Review Letters.
- You can find all our daily Flash Physics posts in the website’s news section, as well as on Twitter and Facebook using #FlashPhysics. Tune in to physicsworld.com later today to read today’s extensive news story on a device that entangles 10 qubits.