
Physicists in Australia have demonstrated how to create an exceptionally stable laser link to send frequency information through the atmosphere. The researchers say that fluctuations in the laser’s frequency are so minuscule that after just a few seconds of averaging such a link could be used to flawlessly transmit timing signals from the world’s most accurate optical clocks. This, they argue, offers the prospect of a global timing network that uses satellites to synchronize optical frequencies between continents.
This ability to connect optical clocks globally could potentially allow physicists to test general relativity, search for dark matter and detect any variabilities in the fundamental constants. It might also be used to improve satellite-based navigation and timing, as well as geodesy – thanks to the effects of gravitational time dilation at varying altitudes.
Optical clocks can now achieve uncertainties about 100 times lower than microwave-frequency, caesium-based atomic clocks – at roughly 1 part in 1018 – while their frequencies have been compared by linking them through optical fibre at distances of up to nearly 2000 km. But extending such comparisons across the globe will be tough, given the huge cost of laying a dedicated fibre with suitable amplifiers. Satellite radio links, on the other hand, are fine for microwave clocks but are orders of magnitude too imprecise for optical timekeepers.
As David Gozzard, Lewis Howard and colleagues at the University of Western Australia in Perth explain in a preprint uploaded to the arXiv server, any link must have a more stable frequency than the optical clocks they connect. Otherwise, the supreme accuracy of those clocks will go to waste. Stability can be raised by averaging a signal over a longer times, but that time is very limited – with some experts anticipating that optical clocks might soon become stable to one part in 1018 after running for just 100 s.
Atmospheric turbulence
The challenge for developers of free-space links is overcoming atmospheric turbulence. Fluctuations in the refractive index along the path of the laser slightly speed up or delay the light’s arrival, leading to phase instability. What is more, turbulence also causes the beam to wander off target and scintillate. This diminishes the beam intensity very briefly but repeatedly, with the loss of signal limiting the averaging time and with it the frequency stability.
To demonstrate how to overcome these problems, Gozzard and colleagues set up a laser transmitter and receiver on the rooftop of their university’s physics department. They then measured the stability of a beam bounced off a corner-cube reflector on another roof 1.2 km away. That 2.4 km horizontal round trip, they say, had about the same level of turbulence as would a link established between the ground and a satellite about 500 km up in a low-Earth orbit.
To maximize the system’s stability, the researchers were able to continually realign the reflected beam using a “tip-tilt” mirror moving in response to the fluctuating output from a photo detector that could capture intensity drops lasting less than a millisecond. In tandem, they used a phased-locked loop together with an acoustic-optic modulator to shift the light’s frequency.
Free-space attempts
This is not the first attempt to stabilize frequency transfers in free-space. The Australian group and colleagues in France reported in January having achieved a stability of 1.6 parts in 1019 after just 40 s of averaging. This compares well with 6 × 10-19 after about 30 h averaging, reported last year by researchers at the National Institute of Standards and Technology in the US when using a 1.5 km open-air link with optical clocks at either end. A group at the Korea Advanced Institute of Science and Technology has also carried out similar measurements on an 18 km open-air link.
However, the latest work pushes up stability significantly. Carrying out their experiment for two weeks in September 2020, Gozzard and colleagues found that the phase stabilisation technology alone allowed a fractional stability of 1 × 10−19 after averaging for a minute. By also stabilizing the amplitude, they were able to reduce signal loss and raise the stability to about 6 × 10−21 after 5 min. As they point out, this happens to be about the length of time that contact can be maintained with a satellite in low Earth orbit.
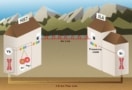
Three top atomic clocks are compared with record accuracy
They then worked out what these figures would mean when linking up with a satellite in orbit. They found that the lower signal bandwidth due to the greater distance would slightly lower stabilities but still keep the system extremely competitive with the best optical clocks. They calculate that frequency comparisons would be limited by the clocks’ own instabilities after just a few seconds of averaging.
To test the technology for real, Gozzard and his colleagues are currently building a 0.7 m telescope on the ground and are hoping to get access to a satellite from either the French space agency CNES or a private company. He points out that they will have to contend with quite severe Doppler shift – a satellite’s movement towards and away from the ground station will cause the incoming signal to abruptly rise and fall by about 10 GHz. But he reckons that experience gained by the team dealing with high-precision microwave shifts on the Square Kilometre Array radio telescope should help them maintain current precision. “We’re confident we can adapt to the Doppler shift,” he says.