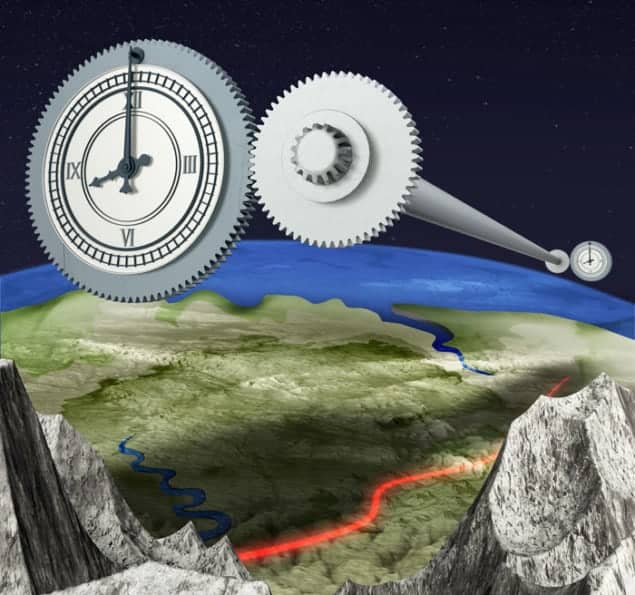
Physicists in Germany have sent a burst of light over a distance of 920 km down an optical fibre – with its frequency remaining stable to the 19th decimal place. As well as supporting the development of highly accurate “optical clocks”, the breakthrough could also be used in a range of commercial and scientific applications including precision spectroscopy, geodesy and very-long-baseline astronomy.
Optical clocks are like conventional atomic clocks but operate at much higher frequencies – about 1015 Hz rather than 1010 Hz. They are therefore much more accurate than atomic clocks, which use a specific electronic transition frequency of an atom as a frequency standard, with the “ticks” being the oscillations between two energy states in an atom. Indeed, the best optical clocks have fractional uncertainties in their frequencies of around 10–18.
But for any clock it is important to be able to compare the frequencies of two or more instruments. In particular, nations set their time standard according to their own atomic clocks, which are compared to others around the world via satellite links to ensure that they all produce the same result. Comparisons are also important for basic research, particularly for testing the fundamental physical laws and constants that are involved in the operation of atomic clocks.
Flying clocks impractical
Unfortunately, satellite links are only able to compare clocks with fractional uncertainties of about 10–15 and therefore cannot be used to compare optical clocks. One alternative being investigated is the use of “flying clocks” that could be shuttled between facilities to compare time. However, unlike atomic clocks, which are amenable to miniaturization, optical clocks tend to be room-sized systems involving lasers and vacuum chambers.
Other groups are looking at how to use commercially available optical fibres to compare clocks. In 2009 Gesine Grosche and colleagues at the PTB standards lab in Braunschweig, Germany, transmitted an optical signal 146 km over a fibre with a fractional uncertainty of 10–19. Then in 2011 Atsushi Yamaguchi of Japan’s National Institute of Information and Communications Technology and colleagues compared two atomic clocks separated by 120 km of optical fibre at a fractional uncertainty of 10–19.
In the new work, a team including Grosche and Katharina Predehl at the Max Planck Institute for Quantum Optics (MPQ) near Munich has sent an extremely stable optical signal 920 km along fibres linking PTB with MPQ. The two fibres run in a buried conduit next to an underground gas pipeline and one is used to send signals from PTB to MPQ and the other to send signals in the opposite direction.
Amplitude and frequency
Predehl told physicsworld.com that transmitting the optical signal over 920 km posed two significant technological challenges. The first was to amplify the relatively weak signal at several points along the route to ensure that it could be measured upon arrival. The second was to ensure that physical disturbances to the optical properties of the fibre – caused by local changes in temperature or vibrations – do not shift the frequency of the signal.
Solving the first problem was relatively straightforward, according to Predehl. This was done by installing nine pairs of erbium-doped fibre amplifiers at nine locations between PTB and MPQ. Although each amplifier introduces some noise into the optical signal, this is distributed symmetrically across the frequency spectrum and therefore does not affect the frequency of the signal, Predehl explains.
Local changes to the optical properties of the fibre can shift the frequency of the transmitted signal and, if left unchecked, would limit the link to a fractional uncertainty of 10–15. The team corrected for these local changes using a feedback loop. Some of the light that arrives at MPQ, for example, is reflected back to PTB – a round trip that takes about 10 ms. This light is then compared with the light that is being sent and the frequency of the latter adjusted so that the two match – the result being that the same frequency is sent and received. By doing this, the team managed to limit the fractional uncertainty of the one-way trip to 4 × 10–19.
Precision spectroscopy
While the link is good enough to compare two optical clocks, that probably will not be its first application – for the simple reason that there is no optical clock at MPQ. Instead, its first practical use is likely to be precision laser spectroscopy, which requires access to an extremely stable frequency standard. While a signal from an atomic clock is currently suitable for MPQ’s spectroscopy research, Predehl says that they are approaching the point where they will need a signal from an optical clock. At that point the lab could use a signal from an atomic clock at PTB for its spectroscopy experiments.
Looking further into the future, Predehl believes that the network could be extended to other labs in Europe. This could allow researchers in standards labs in London, Paris and other locations to compare their optical clocks. Indeed, Giuseppe Marra, who works at the UK’s National Physical Laboratory, calls the PTB–MPQ link an “important step towards a pan-European fibre network for connecting optical clocks”. Indeed, in some European countries, scientists have already taken steps to ensure that they have access to the appropriate fibre networks.
While fibre networks should be able to connect optical clocks within Europe and within Japan, connecting these facilities with those in the US – and even connecting widely spaced labs within the US – could be a challenge. According to Predehl, the technique should work in principle over transatlantic distances – but not on existing links, which don’t have the appropriate amplifiers.
In addition to spectroscopy and metrology, such optical networks could be used in a range of fundamental science. The rate at which an optical clock beats is determined by the value of the fine structure, which should not vary by location or time. Comparing optical clocks in different locations could reveal variations in this constant, which could point to new physics. Optical clocks are also governed by Einstein’s theory of relativity and, for example, tick at slightly different rates when at different altitudes. Comparing clocks could reveal deviations from what is predicted by theory, and also lead to new physics.
The link is described in Science 336 441.