
A glass sphere about 150 nm in diameter has been cooled to its motional quantum ground state using optical tweezers. The feat was performed by physicists in Austria and could bring us closer to testing the macroscopic limits of quantum mechanics. The cooling technique could also lead to experimental investigations of quantum gravity.
In recent decades, researchers have achieved great success in cooling large ensembles of atoms to create exotic systems such as the Bose-Einstein condensate (BEC) – which bagged its creators the 2001 Nobel Prize for Physics. A BEC is an ensemble of ultracold non-interacting atoms that have condensed into a single macroscopic quantum state. Now, physicists want to take this concept much further by cooling solids that comprise much greater numbers of atoms that interact very strongly.
“In a solid, you can cram the same number of atoms you have in an ultracold gas into a volume that’s a billion times smaller,” explains quantum physicist Marcus Aspelmeyer of the University of Vienna, “That provides a natural way of generating large superposition states in which as many atoms as possible are all in one place or another, which is almost impossible in an ultracold gas.”
Experimental challenges
Studying the quantum properties of macroscopic solids has already been done by connecting an object of interest to a nanomechanical oscillator. The object is brought into resonance with the oscillator and the system is cooled by carefully drawing out phonons – which are quanta of vibrational energy. However, there are many experimental challenges associated with this technique, which involves cooling the apparatus to extremely low temperatures.
Another approach is to confine the oscillating object using light in an optical cavity (optical tweezers) and then reduce its vibrations by laser cooling. This minimizes interactions between the object and the outside world – which means that the apparatus does not need to be cooled. Groups including Aspelmeyer’s have attempted this in the past, using one laser to trap the object and a second laser to cool it. However, such schemes have failed to cool large solid objects to their ground states.
In the new work, Aspelmeyer and colleagues used a simpler scheme called cavity cooling by coherent scattering. It was developed in 2001 by team member Vladan Vuletić, who is currently at the Massachusetts Institute of Technology. The technique positions an object at a node of a standing wave field created by a single laser in an optical cavity. The benefit of this set-up is that photons cannot elastically scatter off the object.
Stokes versus anti-Stokes
There are, however, two much weaker inelastic scattering processes that can occur. One is Stokes scattering, which transfers energy from a photon to the object resulting in a lower-energy photon. The second process is called anti-Stokes scattering and involves energy being lost by the object, thereby creating a higher-energy photon. By judiciously selecting the trapping frequency, the researchers suppressed the Stokes scattering while maximizing the anti-Stokes scattering, thereby removing energy from the object and cooling it.
“When we applied this cooling method to our system, it immediately increased the cooling rate by a factor of ten or larger,” says Uroš Delić of University of Vienna, who is lead author on a paper describing the work. “It allowed for three-dimensional cooling rather than just one and basically solved all the problems we were having with our previous setup,” he adds.
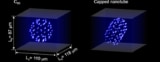
Engineered atomic arrays for quantum computers
After much laboratory work, they successfully cooled a glass nanoparticle containing about 100 million atoms to its motional ground state. Whereas schemes that use mechanical contact for cooling invariably require cryogenic cooling, the current experiment was performed using equipment at room temperature.
The researchers now hope to utilize an isolated glass sphere in its motional ground state to perform manipulations that would otherwise be difficult or impossible. “With laser light, we can arbitrarily change the potential landscape,” says Aspelmeyer, “If a particle in a harmonic ground state sees a non-linear potential, we will automatically generate a non-classical state. These are things that you fundamentally cannot do with clamped solid-state oscillators.” Ultimately, the researchers would like to study objects large and dense enough to generate a detectable gravitational field.
James Millen of King’s College London is enthusiastic about the results. “This is the first step towards doing something like the quantum double slit experiment with something truly massive,” he says. “That would tell you whether or not quantum mechanics works on this mass scale. There are all sorts of reasons people think it might not and all sorts of theoretical modifications to quantum mechanics that would be ruled out if you could do that experiment. However, you cannot do any experiment testing quantum mechanics until you’ve done this first step of cooling the particles down to their motional ground state.”
The research is described in Science.