Martin Green – Scientia Professor at the University of New South Wales, Sydney, and Director of the Australian Centre for Advanced Photovoltaics – talks to Anna Demming about developments in the physics and politics around solar cells over the past three decades that have helped and hindered photovoltaic energy suppliers to finally outbid fossil fuels.

In a recent perspective article you highlight potential production of a terawatt per year from photovoltaics worldwide within the next decade. What is the significance of a terawatt per year?
The world is emitting 40 billion tonnes of CO2 a year, but the thinking is we’ve got a budget of 800 billion tonnes maximum. So you’ve got 40 years to cut 40 billion tonnes out if you do it linearly – 1 billion tonnes a year. One terawatt from photovoltaics will reduce it by about one and a half billion tonnes of CO2 a year if you’re displacing coal from electricity generation or oil from transport. So without doing anything else solar will put you on that trajectory, as long as you’re displacing those two components, which account for a huge fraction of the total CO2 budget.
Once you’ve displaced all the coal from electricity generation and oil for transport, you’ve got to start displacing carbon emissions from industrial processes and things like that, which is a bit more challenging, but you’ve got a little bit of time to work on that.
How did you get started in solar cell research?
I did my undergraduate degree in electrical engineering back in the late sixties. Microelectronics was a really exciting area because they were just starting to put multiple transistors on chips and things like that. But I got sort of disillusioned with that area because I’d missed the whole computer revolution and everything that followed on subsequently from microelectronics, and then I realized that my training would put me in a good position to get involved in photovoltaics. It was around the time of the oil embargoes of the 70s, and new sources of energy were attracting more interest.
I started working on a tunnelling structure during my PhD, in Canada under a Commonwealth scholarship. The structure had a metal separated from a semiconductor by an insulator, which is quite a common structure in microelectronics, except here the insulator was made very thin so that electrons can tunnel through it. My thesis was looking at what type of microelectronic application you might be able to use this structure in, but just having an insulator on the surface of the semiconductor, improved the surface properties of the semiconductor. And that turned out to be what was holding back solar cell performances.
What helped you establish such a successful group in this field?
Studying that first structure gave us a unique approach to improving the cell performance. We were a fairly small group then, but we were getting world record voltages, better than other groups that were being supported quite generously in the late seventies. When Jimmy Carter was president, there was actually more money going into photovoltaic research in the US than there is these days, even if you discount the depreciation in the value of the dollar.
We were able to set up a reasonably large group by Australian standards, but in 1983 we got our first world record for the cell performance. In the solar cells field, these are not just what you measure, you send it to a recognized test centre and have the performance confirmed there so that the result can’t be disputed. That helped with research funding because it was something quantifiable that everyone could understand.
1983 is a long time ago – was it hard to maintain that momentum?
After Carter, Reagan was in power and he didn’t like anything to do with renewables, so he just scrapped all the programs and all our US competitors sort of disappeared one by one. We managed to maintain our funding right through the 1980s until Chernobyl in 1986. That then reinvigorated interest in alternative energy sources, particularly in Europe.
We maintained continuity right from the 70s through to the late eighties, which didn’t happen in other countries. There are probably only three groups worldwide that maintained that continuity with a reasonably large size program, and they’re the three groups that are probably the most highly regarded worldwide in terms of solar research these days. All through that period we kept increasing the performance of our cells and setting new world efficiency records, which was again good for our funding here and kept us highly motivated.
There were very good students too because in the 80s in Australia, the scholarship stipends were very low and so on, so only those really dedicated to the field that they wanted to study did postgraduate research. Being in the solar area, we got some very motivated highly qualified and credentialed students into our programs. That also helped us make progress, having such a strong group of people together.

What key breakthrough has helped improve solar cell efficiency?
In a solar cell, light creates excited electrons within the material, but they relax back to their ground state in typically microseconds. So you haven’t got long to collect them or extract them from the cell. You get the maximum voltage from the cell when the rate of generation of the electrons by light balances the rate that they relax back, and as you increase the voltage of the cell, they start relaxing back more quickly. And if you have bad surfaces, they relax back even more quickly.
Our key breakthrough was the passivated emitter and rear cell known as the PERC cell. My first drawing of the PERC cell was in 1983. Passivated in this area means – reducing the electronic activity, so stopping the excited carriers from relaxing back. The tunnelling structure had allowed us to control what was happening at the surface of the cell. Solar cells use terminology that was originally developed for transistors. So the emitter is the top region of the cell. So all it means is we fixed up the surfaces on both sides of the cell. We fixed up the top and made our first efficient PERC solar cell in 1988. This was the first time we successfully achieved proper passivation on both surfaces, and that was the vehicle for taking us through to 25% efficiency in 2008, which is still the record for a PERC cell.
What impact have these PERC cells had in industry?
The fraction of world manufacturing solar cell capacity that was PERC by the end of last year was 60% – that all came online during the year. Only 35-40% of the product produced last year was PERC so this will be the first year that it’s the dominant technology used commercially.
The previous technology had been used for 40 years before that, so it’s a really big change in the industry, which has really given the efficiency a bit of a boost. There’s been a round of creativity associated with the change that’s really quite striking.
What led to the current technology persisting for so long?
The standard technology was improving incrementally, but then everyone could see that we were running out of room to move. So some of the companies started investigating PERC technology and started producing results where the cells were markedly better and so on. And then the whole industry transferred over to that new technology. The transition all happened over just five years. When the industry decides it’s time to move change can be very rapid.
Other high efficiency approaches such as rear contact structures have an additional complication in that they need a different type of silicon wafer from what has been traditionally used. That creates an additional barrier that the PERC cell didn’t have so it could take a little longer to transition because all the supply chains need rejigging. So I feel pretty confident that PERC will have a 10 year reign. But by the end of that, people will have got to our 25% efficiency or maybe beyond and they will be looking for ways to get 27% efficiency.
Is energy storage an issue in terms of the market reaching a terawatt a year?
It’s going to be important to develop storage, but the cost of lithium ion batteries have been coming down nearly as quickly as photovoltaics – about 20% per year reduction. So that’s been very timely.
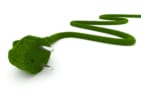
Energy storage technologies vie for investment
In Australia, there’s a lot of interest now in pumped hydro storage. One of my former students (Andrew Blakers) who’s now at ANU has been doing studies of off-river pumped hydro where you make a swimming pool at the top and bottom of a mountain, and just pump the water uphill – you’re not relying on having a river flowing and dams and all that kind of stuff. He’s identified 22,000 suitable sites around Australia to store essentially all Australia’s electricity for a day or more. And, he figures you only need 22.
He’s now extended his study to the whole world and found hundreds of thousands of sites where this type of storage could be put in. There are already many working systems like that around the world, and it’s a lot simpler and more environmentally benign than the standard pumped hydro systems. That’s traditionally been the way electricity has been stored in the past and it’s still the cheapest way of storing huge quantities of electricity.
So the end of the world is not nigh?
The cost reductions in photovoltaic, occurred quite quickly, really just over the last 10 years. A lot of our grid utilities are now buying solar electricity under power purchase agreements – someone just bids to supply electricity at a predetermined rate over a 20 year period or something similar. Some of the bids that were coming in for solar supply from 2016 onwards have been really very low. That really was a turning point for me. Once you saw these bids for solar supply undercutting the normal costs of electricity generation by such large margins, you realized it could more than compete with the traditional suppliers.
Traditional ways of doing things, like generating electricity from coal, have become very cheap but if you look at the process, it’s really a very complex process involving many stages. It’s just an example of technology lock in – it’s got so refined that the costs have become cheap and made it difficult to be displaced, but with the added impetus that comes from trying to control CO2 emissions, solar technology has been driven to the stage that it’s now reflecting the natural order of things in terms of the costs you’d expect from the two different technologies.
At the wholesale level in Australia our electricity generation is only 1% of GDP; at the retail level that’s probably 3% of GDP, which is quite small considering it makes up more than 30% of CO2 emissions. So it’s having a disproportionate impact – politically as well. Here in Australia we’ve lost about five prime ministers over the issue. Fortunately the economics now well and truly favour solar. I think we’ll see fairly rapid phasing out of coal generation in Australia despite the wishes of our present bunch of politicians.
Future solar cell technologies
What might supercede PERC solar cells?
The highest efficiencies achieved so far are with back contact structures. In the traditional cell, the positive and negative contacts are on opposite surfaces, but back-contact structures have them both on the rear. Most of the carrier-generated excitation occurs very close to the front surface – within 2 micrometres of a 180-micrometre thick cell. So in order to maintain the electron in the excited state for sufficient time to get it to the back of the cell where the contacts are, you have to have very high-quality silicon material, much better than is available cheaply commercially. The other issue is that the processing to get both contacts on opposite surfaces is a lot more complex. So that means the approach is fundamentally more expensive.
However that could well be the way the industry goes. In microelectronics you get these continual improvements through reducing the size of the components and so on, but in the solar field, you get continuous reductions in the actual cost of making the cell through economies of scale and streamlining the processing and all that kind of thing. You’ve got a lot of other things like glass and aluminium frames, and it is more difficult to get the costs down for these components because they are such mature products. But by improving module efficiency you can generate the same energy with fewer modules, and so use less of these components. So the industry is pushing to more and more sophisticated cell structures to get higher efficiencies. PERC will probably prevail for at least 10 years, but if we’re not successful in implementing our (tandem cell) ideas after that it’ll probably go to one of the rear contact structures as the mainstream for the industry.
What about alternatives to silicon?
For spacecraft they stopped using silicon solar cells at the turn of the century and they’re using cells based on gallium arsenide (GaAs). The advantage there is that you can stack cells of different compositions on top of one another very easily in the GaAs system – to make tandem solar cells. If you can stack cells of different material on top of one another, you can design the upper most cells so that they convert the blue light very efficiently, and a cell underneath like a silicon cell that converts the red light well, and you end up with a better overall conversion efficiency. But it gets very expensive because you have to grow GaAs on high-quality germanium wafers, and germanium is much scarcer than silicon. The process is also more complicated.
The other material used commercially is cadmium telluride, which isn’t a great choice because cadmium’s one of the more toxic metals. And then the other component of the material, tellurium, is a material that is as scarce as gold. Then there is CIGS – copper indium gallium diselenide – where there is a bit of an issue with the supply of indium. So silicon is by far the best choice of these.
With silicon you grow a crystalline ingot and then you slice it into wafers. These other technologies you can deposit directly as thin films, so that’s their advantage. Until the early part of this century, it was thought that the thin films just had to be cheaper than silicon. But with the scaling up of the industry, the cost of making the wafers has dropped really dramatically. So the glass and frame cost more than the silicon wafers now.
Will perovskites ever make it in commercial solar cells?
Perovskites could be an interesting technology in the future. It’s very well matched to silicon – they respond really well to blue light and the silicon responds very well to red light, so they’re a good combination for the tandem cell approach. Oxford PV are leading in commercializing perovskites and that is what they are looking at.
But of the seven different cell technologies that have got to over 20% efficiency, silicon is by far the most stable, and perovskite is by far the most unstable. Perovskites are a really hot topic in physics. There are thousands of people who work in the area, but none of the published work is showing that the stability is under control. Oxford PV, of course, aren’t publishing too much about exactly what they’re doing. So, maybe Oxford PV is doing something a lot better than the thousands of other teams that are working on it, though that seems challenging to me.
Martin Green’s perspective article “Photovoltaic technology and visions for the future” is available in the recently launched journal Progress in Energy.
- This interview was edited 31 July 2019 to amend the maximum CO2 budget and the number of off-river pumped hydro sites needed for Australia.