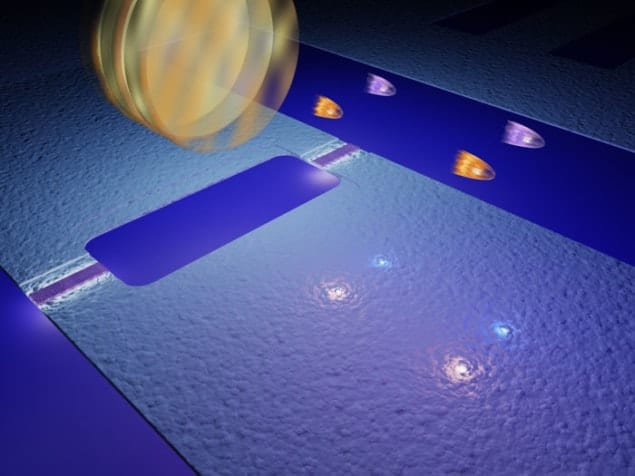
Quantum mechanics tells us that the vacuum is not empty but is filled with virtual particles that pop into and out of existence. Normally these particles are hidden from our view, but now a team of physicists has used the electrical equivalent of an ultrafast mirror to convert virtual photons into real electromagnetic radiation. Known as the dynamical Casimir effect, it was first predicted more than 40 years ago.
The static Casimir effect, put forward by Dutch physicist Hendrik Casimir in 1948, involves two perfectly reflecting parallel mirrors that, when placed in a vacuum, will be attracted to one another. This attractive force is caused by the radiation pressure exerted by virtual photons outside the mirrors and the fact that this pressure exceeds the pressure between the mirrors because of the limited number of modes of electromagnetic vibration that are permitted within this gap. In other words, the force results from a mismatch of electromagnetic modes in space.
The dynamical effect was proposed by Gerald Moore in 1970 and is caused by a mismatch of modes in time. The phase of an electromagnetic wave goes to zero at the surface of a mirror, if that mirror is a perfect electrical conductor. When the mirror is moved slowly through a vacuum, this zero point can move with the mirror. However, if the mirror is moved at a significant fraction of the speed of light, then the electromagnetic field does not have time to adjust but instead becomes excited and as a result generates real photons. Put another way, the mirror prises virtual photons (always produced in pairs) apart so that instead of rapidly annihilating, the particles are free to remain as real photons.
High-speed challenge
The static effect has been observed in many experiments carried out over more than a decade, whereas confirmation of the dynamical version has until now proved elusive, partly because of the challenges involved in moving a mechanical object at such high speeds. Christopher Wilson of Chalmers University of Technology in Sweden and colleagues have managed to get round this problem by rapidly varying the electrical properties of a mirror rather than moving it in space.
The researchers place a tiny device used for measuring extremely weak magnetic fields – a superconducting quantum interference device (SQUID) – at one end of an electrical transmission line. The idea is that the SQUID reflects the electromagnetic waves associated with virtual photons, with its inductance determining how imperfect a mirror it is. This imperfection is related to how large (i.e. different to zero) the waves’ phase is at that point. Applying a varying magnetic flux across the mirror causes its inductance to oscillate, which allows the researchers to vary the extra distance that the waves need to travel beyond the mirror before their phase falls to zero. “It turns out that the equations are exactly the same as moving a physical mirror along that distance,” explains Wilson.
Quarter the speed of light
By switching the magnetic flux billions of times a second, the researchers made the mirror vibrate at up to a quarter of light speed. As a result, they were able to detect microwave electromagnetic radiation at the far end of the transmission line. The radiation has the properties expected of photons produced via the dynamic Casimir effect. Its frequency is about half that of the mirror’s oscillation frequency and the relationship between the strength of the magnetic flux and the intensity of the measured radiation is broadly in line with theoretical predictions. The team also found that the flux strength–intensity spectra contained duplicated patterns of noise, which is strong evidence that photons are being produced in correlated pairs.
While the research was not aimed at practical applications, Wilson says that their apparatus might conceivably provide a way of generating entangled photons for experiments in quantum information processing. He also believes that the work could help advance fundamental physics, pointing out, for example, that the Hawking radiation believed to be emitted by black holes involves the production and then splitting of pairs of virtual photons, one of which falls into the black hole while the other is then emitted as a real photon.
“Beautiful experiment”
John Pendry of Imperial College London, who is not a member of the team that carried out the research, describes the demonstration by Wilson and colleagues as “a beautiful experiment that explores in an entirely novel way the delicate features of Casimir forces” but adds that “the whole argument turns on whether you believe that wobbling the parameters of a SQUID really is identical to wobbling a real mirror”.
Meanwhile, Giuseppe Ruoso of the INFN National Laboratory of Legnaro in Italy says that “if the result is confirmed by other independent measurements, it will mean another great step in understanding the nature of the vacuum, yet again confirming the validity of quantum mechanics”. Ruoso is part of a group of physicists in Italy that is also trying to observe the dynamical Casimir effect, but doing so using repeated laser pulses to periodically vary the reflectivity of a slab of semiconductor inside a microwave cavity. “I don’t see any practical applications on a short timescale, but I’m sure there will be some in the future given that the vacuum is the most abundant ‘element’ in our universe.”
The research is reported in Nature.