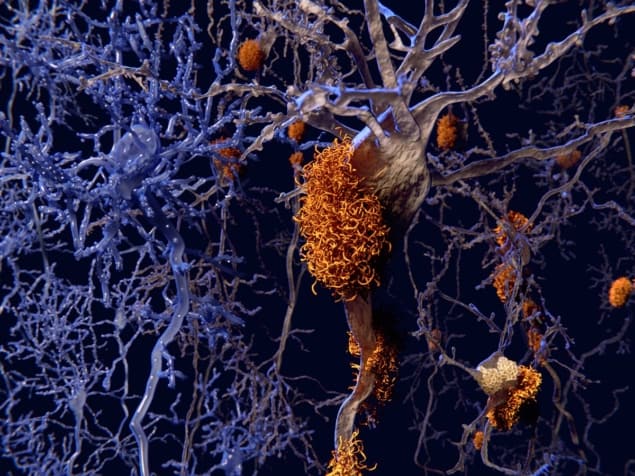
Amyloid fibrils associated with some brain diseases have been detected in tissue using new optical techniques developed by researchers in France. The team used a near-infrared signal from the fibrils to identify the presence of amyloid structures in the brains of live mice and believe that their method could form the basis of a clinical test for neurodegenerative diseases. The scientists have also developed an imaging technique that monitors the emission of blue light from amyloid fibrils and used it to study human tissue samples.
Amyloid fibrils are nanowire-like structures that self-assemble from proteins. They occur in the human body, where they can clump together to form larger deposits called amyloid plaques. The presence of these plaques in the brain is associated with medical conditions such as Alzheimer’s and Parkinson’s diseases and deposits of amyloid fibrils elsewhere in the body are associated with diabetes. Despite being linked to several serious diseases, the reasons why these fibrils form and make deposits are still poorly understood. Furthermore, detecting fibrils in living patients is extremely difficult.
“The proteins involved in these amyloid deposits are different depending on the disease,” explains biophysicist Vincent Forge of the Biosciences and Biotechnology Institute of Grenoble. “Most of the time one protein is related to one disease but the tau protein, for example, is related to both Alzheimer’s and Parkinson’s disease.”
Labelling woes
Researchers would like to understand exactly what makes these proteins self-assemble into amyloid fibrils and subsequently amyloid plaques. They normally do this by labelling the amyloid proteins with specific dye molecules such as thioflavins, but this makes interpreting results difficult: “Most of the experiments in vitro are about the kinetics of amyloid plaque formation,” explains Forge. “It’s important to be able to do these experiments without thioflavin because we don’t know whether or not the label changes the kinetics we’re trying to characterize.”
When illuminated by near-ultraviolet light, most proteins emit weak blue light. Recently, several research groups have reported that this emission becomes much stronger when the proteins self-assemble into fibrils, for reasons that are still debated. “What has been done previously has mainly been on amyloids prepared in vitro or on cellular experiments,” says Forge. “We are the first to show detection of amyloid deposits within real human samples.”
The team imaged the structure of plaques at the micron scale without staining the tissue. This could prove invaluable for studying how amyloid plaques form in tissue, which could help to develop new drugs to tackle diseases associated with the plaques.
Diagnostic window
While the technique has proven useful, it is hampered by the fact that UV and blue light travels very short distances in tissue – which means that images cannot be taken within bulk tissue samples. Now, Forge and colleagues report that amyloid fibrils illuminated with near-infrared radiation emit a dim, near-infrared signal. This signal could be especially valuable, say the researchers, because both excitation and emission wavelengths fall within the “diagnostic window” to which tissue is relatively transparent.
“It’s just an observation at present,” says Forge, “We think it’s caused by inelastic scattering of the light from the object we detect, but we don’t know exactly the origin of this phenomenon.” Nevertheless, the researchers were able to show that, when they added a chemical to depolymerize the amyloid fibrils, the infrared signal vanished.
Indeed, they have also used the near-infrared technique to detect amyloid plaques within the brains of anaesthetized mice that were genetically engineered to develop Alzheimer’s disease. In the future, they suggest, the technique could potentially detect the disease in humans. Today, Alzheimer’s disease is usually diagnosed by assessing cognitive symptoms in a patient.

Super-resolution imaging provides insight into Alzheimer’s disease
Medical physicist Steen Madsen of the University of Nevada says that the infrared signal could be useful for preclinical studies: “In mice and rats, where the brains are pretty small, you certainly would be able to monitor therapeutic interventions to see whether they have any effect,” he says. He is more sceptical about clinical value as the tissue penetration depth of near-infrared radiation is no more than 1 cm: “I don’t see how it would work in humans unless you could open up the brain and put a probe in there. There are other techniques such as PET scanning that are much more expensive and complex, but which would certainly be preferable from a non-invasive point of view,” he says.
Forge acknowledges these difficulties and says “It’s not for tomorrow because it will necessitate further technological advances.” He says, however that “we have plans”, but did not give further details.
The researchers also believe that the optical properties of amyloid fibrils could be used to create biophotonic devices.
The new technique is described in Nature Photonics.