
Individual polyatomic molecules have been trapped in arrays of optical tweezers for the first time. Researchers in the US were able to control individual quantum states of the three-atom molecules and the technique could find applications in quantum computing and searches for physics beyond the Standard Model.
Cooling molecules to temperatures near absolute zero is an exciting frontier in ultracold physics because it provides a window into how chemical processes are driven by quantum mechanics. For decades, physicists have been cooling atoms to ultracold temperatures. However, molecules are much more challenging to cool because they can hold energy in many more degrees of freedom (rotation and vibration) – and cooling a molecule requires removing the energy from all of these. Considerable success has been achieved with diatomic molecules, but the number of degrees of freedom grows steeply with every additional atom, so progress with larger molecules has been more limited.
Now, John Doyle, Nathaniel Vilas and colleagues at Harvard University have cooled individual triatomic molecules to their quantum ground states. Each molecule comprises a calcium, an oxygen and a hydrogen atom.
Linear geometry
“The main thing that we like about this molecule is that, in the ground state, it has a linear geometry,” explains Vilas, “but it has a low-lying excited state with a bent geometry…and that gives you an additional rotational degree of freedom.”
In 2022, a team including Vilas and Doyle laser cooled a cloud of these molecules to 110 μK in a magneto-optical trap. No one, however, has ever previously cooled individual molecules containing more than two atoms to their quantum ground states.
In the new work, Vilas and colleagues loaded their molecules from a magneto-optical trap into an array of six adjacent optical tweezer traps. They used a laser pulse to promote some of the molecules to an excited state: “Because this excited molecule is there there’s a much larger cross section for the molecules to interact,” says Vilas, “So there’s some dipole-dipole interaction between the ground state and excited state, that leads to inelastic collisions and they get lost from the trap.” Using this method, the researchers reduced the number of molecules in almost all the tweezer traps to just one.
Before they could proceed with imaging the molecules, the researchers had to decide what wavelength of light they should use for the optical tweezer. The central requirement is that the tweezer must not cause unintended excitation into dark states. These are quantum states of the molecule that are invisible to the probe laser. The energy structure of the molecule is so complex that many of the high-lying states have not been assigned to any motion of the molecule, but the researchers found empirically that light at a wavelength of 784.5 nm led to minimal loss.
Population accumulation
The researchers then used a 609 nm laser to drive a transmission from a linear configuration of the molecule in which the three atoms are in a line, to a vibrational mode in which the line bends. The molecules were left in a combination of three near-degenerate spin sublevels. By subsequently pumping the molecules with a 623 nm laser, they excited the molecules to a state that either decayed back to one of the original sublevels or to a fourth, lower-energy sublevel that did not absorb the laser. With repeated excitation and decay, therefore, the population accumulated in the lower sublevel.
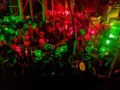
Laser cooling of polyatomic molecules brings ultracold chemistry into the spotlight
Finally, the researchers showed that a small radio-frequency magnetic field could drive Rabi oscillations between two energy levels of the system. This could be hugely important for future research in quantum computing: “The geometry doesn’t have any bearing on this current work…We have these six traps and each one is behaving completely independently,” says Vilas. “But you can think of each one as an independent molecular qubit, so our goal would be to start implementing gates on these qubits.” It could even be possible to encode information in multiple orthogonal degrees of freedom, creating “qudits” that carry more information than qubits.
Other possibilities include searches for new physics. “Because of the diverse structure of these molecules there’s coupling between the structure and different types of new physics – either dark matter or high-energy particles beyond the Standard Model, and having them controlled at the level we have now will make the spectroscopic methods way more sensitive,” says Vilas.
“It’s sort of a milestone in the field because it says we can control even single molecules that have more than two atoms,” says Lawrence Cheuk of Princeton University in New Jersey; “If you add a third atom, you get a bending mode, and this is very useful in certain applications. So in the same work, the Doyle group not only showed they can trap and detect single triatomics: they also showed that they can manipulate in a coherent manner the bending mode inside these triatomics.” He is intrigued about whether still larger molecules can be manipulated, opening up the study of features such as chirality.
The research is described in Nature.