
The distribution of pressure inside the proton has been mapped by physicists using the electron accelerator at Jefferson Lab in Virginia, US. By putting a new twist on Compton scattering, the team showed that the extreme repulsive pressure experienced by quarks in the centre of the proton is balanced by strong attractive forces around the edges. The research sheds further light on how quarks are confined inside protons and other hadrons – which is one of the most important mysteries of modern particle physics.
Quantum chromodynamics says that the strong nuclear force binds quarks into protons through the exchange of force particles called gluons, much as the electromagnetism binds electrons into atoms by the exchange of photons. Whereas electromagnetism gets weaker with increasing distance, the strong nuclear force gets stronger. Therefore, knocking a quark out of a proton and studying it in isolation is impossible. Instead, striking a single quark too hard gives the gluon field so much energy that a new quark-antiquark pair is created: “You would produce either a neutron plus a meson (a bound state of a quark and an antiquark) or a proton plus a meson,” explains Jefferson Lab’s Volker Burkert. “We want to study the transition from a proton to a proton so we can see what’s happening in the proton itself – not in the transition to some other state.”
In 1996, Xiangdong Ji of the University of Maryland proposed a way to do this using a process called “deeply virtual Compton scattering”. This involves firing high-energy, spin-polarized electrons at protons. Ji realized that such an electron could exchange energy and angular momentum with a proton in the form of a high-energy virtual photon, which only exists for a tiny fraction of a second. The proton then releases some of this extra energy in the form of a real photon. Ji showed theoretically that, if the scattered electron and proton and the emitted photon were all detected, this could reveal the pressure inside the proton.
Essential developments
Deeply virtual Compton scattering was first detected experimentally in 2000 by Burkert’s group at Jefferson Lab and, independently, by physicists in Germany. Subsequently, there have been several essential experimental and theoretical developments by Burkert, Ji and many others such as measurements of the probability that the process will occur (the scattering cross section) for spin-polarized electrons of various energies and calculations of the links between scattering and internal pressure.
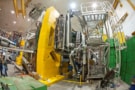
Proton’s weak charge is measured to high precision
In the new research, Burkert and colleagues at Jefferson Lab brought all of this together for the first time in an experiment. They measured deeply virtual Compton scattering between a spin-polarized beam of 6 GeV electrons and a cryogenic liquid hydrogen target to probe the internal structure of protons. They calculated that there was a repulsive pressure in the proton’s centre of about 1035 Pa – greater than in the centre of a neutron star. Around 0.6 fm (0.6 x 10-15 m) from the centre, however, the forces became strongly attractive and peaking at 0.8 fm radius. The researchers say that this is a significant improvement over our previous understanding of the pressure inside the proton. They anticipate it should be possible to reduce their errors further following the Jefferson Lab accelerator’s recent upgrade from 6 GeV to 12 GeV, potentially unlocking the secrets of quark confinement. The researchers also intend to explore deeply virtual Compton scattering in neutrons.
“It’s a good, interesting study and a first step into the extraction of the quark pressures inside a proton,” says particle physicist Kresimir Kumericki of the University of Zagreb in Croatia. He wants more technical details of the researchers’ error analysis but he says that “even if the uncertainties are larger [than they claim], it’s still important that…such analysis can be used to get a physical picture of the proton.”
Universal concept
“I think it’s a really interesting generalization of the theoretical concept of pressure,” says Oleg Teryaev of the Joint Institute for Nuclear Research in Dubna, Russia. “Pressure unites all the scales, from the universe and cosmology – where you have the cosmological constant – to stars, to hydrodynamics, to heavy-ion physics and now finally to protons. All elements of this relation can be studied and I am looking forward to that with excitement.”
The research is described in Nature.