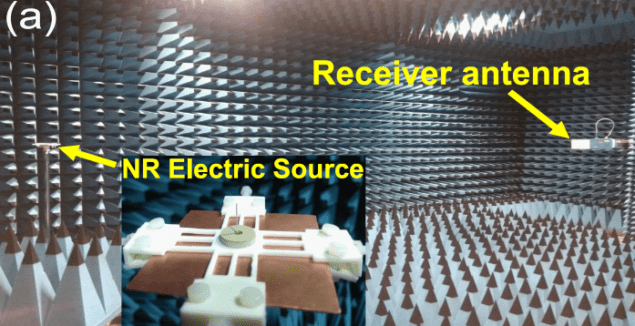
An international team of physicists has created what might seem like a contradiction in terms: a non-radiating source of electromagnetism. By placing antennas inside a hollow dielectric disc, the researchers exploited interference phenomena to create a non-radiative “meta-atom” that could have applications in several areas, including sensing and wireless power transfer.
Maxwell’s equations tell us that accelerating charges radiate energy in the form of electromagnetic waves. This idea has led to the development of much modern technology, most notably radio communications in all its various guises. But it also posed a challenge to early 20th century physicists seeking to explain the stability of atoms, given that electrons were thought to follow (curved) orbits around atomic nuclei and therefore continuously lose electromagnetic energy.
The response to that problem was Niels Bohr’s atom and the development of quantum mechanics – in particular, the idea that electrons can only occupy certain discrete energy levels. Beyond the quantum world, however, some physicists wondered whether it might be possible to create classical “meta-atoms”: macroscopic objects containing alternating currents that confine the electromagnetic energy they generate and so prevent emission to the far-field.
Electric moments without any poles
This idea received a boost in 1957 when the theoretical physicist Yakov Zel’dovich predicted that the effects of parity violation on electric charges would yield electric moments without any poles. These so-called anapole states were discovered in the late 1990s when researchers at the JILA centre in the US made measurements of parity non-conservation in caesium atoms. Since then, other physicists have suggested that these anapole states could help explain how dark matter remains hidden electromagnetically.
In practical electrodynamic terms, anapoles can be generated by precisely tailoring charge distributions in time and space. These distributions are represented as a series of point-like electric and magnetic multipoles, the emissions from which interfere destructively in the far-field, leaving electromagnetic energy confined within a small space around the sources.
The simplest way to generate anapoles is to superimpose the fields from an electric dipole and a toroidal dipole – the latter can be imagined as electric currents flowing around the cross section of a torus. This has been demonstrated experimentally several times over the last few years, with various research groups exploiting nanofabrication techniques and metamaterials to observe anapole states at different frequencies – from microwaves to the visible range.
Anapole observations
In the new work, Esmaeel Zanganeh and Polina Kapitanova at ITMO University in Saint Petersburg, together with colleagues in Russia, Germany, Australia and China, have put together a relatively simple system to observe both electrical and magnetic anapole states – something not achieved before. The set-up consists of a radiating antenna placed inside a thick, hollow disc shaped like a roll of sticky tape. The disc is made from a dielectric with extremely high permittivity, which allows the disc to be smaller than the wavelength of the radiation it emits. The idea is that the electromagnetic waves from the antenna interfere destructively with secondary waves produced by electric current induced in the dielectric – thereby yielding a non-radiating source.

The researchers first modelled their system numerically, assuming a 25 mm-long copper electric dipole antenna emitting radio waves and placed along the thickness of a 20 mm-diameter dielectric disc. Varying the frequency of the antenna, they compared the output of this source with that of the same antenna operating in free space. They found that at the disc’s resonant frequency (375 MHz), the system radiated slightly more power than the antenna alone, but that at a slightly higher frequency (411 MHz) the system’s radiated power dropped to seven orders of magnitude below that of the naked antenna.
Backing their modelling up with analytical calculations, the researchers showed that the sharp drop in power resulted from the antenna and disc generating equal and opposite radiation fields at 411 MHz, leaving just the confined electromagnetic field inside the source. They also did similar calculations involving a magnetic loop antenna – one that intercepts the magnetic field of electromagnetic waves – in place of the electric dipole device. Here, too, they found that by tuning the antenna away from its resonant frequency they could reduce the radiated (magnetic) power to near zero, and about three orders of magnitude lower than that of the antenna alone.
Finally, Zanganeh and colleagues tested their system experimentally, albeit at microwave rather than radio frequencies due to difficulties with materials and testing facilities. By placing a 6.4 mm-diameter disc containing an 18 mm long antenna in an anechoic chamber and using a horn antenna to pick up its far-field emissions, they measured a radiation pattern that closely matched their simulations.

‘Metasheet’ blocks a narrow band of radiation, letting the rest pass
The researchers believe that their new “meta-atoms” could have several practical applications. In particular, the very small radiative output of such devices, coupled with a larger concentration of electromagnetic energy in their immediate vicinity, could see them used for sensing, radiofrequency identification or near-field wireless power transfer, they say.
The research is published in Physical Review Letters.