
Scientists generated the first petawatt laser pulse in the 1990s. In the decades that followed, lasers that produce petawatt-level power were built – equivalent to one quadrillion (1015) watts, or a significant fraction of the energy Earth receives from the Sun in a short amount of time.
One potential application of petawatt laser technology is advanced ion accelerators for particle therapy. Ongoing research has been dedicated to myriad topics in this area, from increasing particle energies and yields to improving beam quality and control.
Renewable targets are also on scientists’ radar.
Laser-driven acceleration works by firing extremely powerful laser pulses at targets made of thin metallic foil. The heat generated ejects electrons in the material, while the heavy atomic nuclei remain in place, creating a strong electric field that can then then launch a pulse of protons.
But conventional metallic foil targets present two challenges for applications of laser-accelerated ions. First, intense laser pulses damage targets, so they need frequent replacement – making it difficult to generate several ion pulses per second. Second, with each shot of the laser, debris is generated and accumulates on the laser optics, reducing the quality of the laser pulse. With foil targets, ions are then accelerated from a contaminated layer containing a mix of different hydrocarbons, making particle acceleration difficult to control.
Cryogenic hydrogen jets may supply an alternative. These targets, which have been explored for inertial confinement fusion and other research studies, can be used to generate proton beams without being replaced as frequently as metallic foils. Their performance as proton sources to date has been limited to low (with respect to therapeutic applications) particle energies and yields, but current designs offer a continuous jet of pure hydrogen that, a recent proof-of-concept experiment suggests, might exceed the performance of metal foils.
An international group of scientists led by researchers at Helmholtz-Zentrum Dresden-Rossendorf (HZDR) is investigating micron-sized cryogenic hydrogen jet plasma as an alternative to metallic foil targets. The plasma filament renews itself, so the petawatt laser has a new target for every shot.
“From the beginning, it was clear that this type of target had some unique advantages which you could not easily find elsewhere,” says Martin Rehwald, a postdoctoral researcher at HZDR.
HZDR scientists first reported on laser-accelerated protons from cryogenic hydrogen jets in 2017 (in Scientific Reports, Physical Review Letters and Applied Physics Letters). Their most recent study, published in Nature Communications, describes different acceleration schemes for their petawatt laser-cryogenic target system.
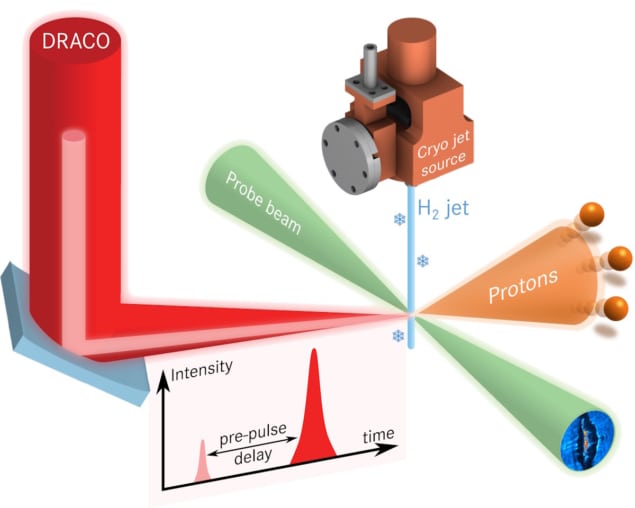
Hydrogen, liquefied in a cryogenically cooled copper box, is pressed through a micron-sized aperture into a vacuum, where evaporative cooling sets in to form a solid target. Laser-accelerated protons are produced when a high-intensity laser beam hits this cryogenic target, with radiation pressures pushing electrons out of the hydrogen and creating the extreme electric fields needed to accelerate protons.
The HZDR team’s research demonstrated that priming the cryogenic hydrogen jet with a weaker light pulse before the main pulse yields a two-fold increase in proton energy (up to 80 MeV) compared with the non-primed case. The weaker pulse allows the hydrogen filament to expand – and the acceleration distance to increase – before the main high-intensity pulse hits the jet.
Simulations suggest that proton energies exceeding 100 MeV can be expected when experimental conditions, including the target density profile, are optimized.
“We know from simulation how to further increase the proton energies. Here, the hydrogen content of the target actually allows us to model the interaction more precisely with respect to metallic foils,” says Rehwald. “You can easily imagine [acceleration schemes that] lead to higher particle energies than just having a stationary [electric] field. But in order to reach such regimes, we need to match our laser beam and the density profile very precisely. All of this can only be done with great control of the target.”
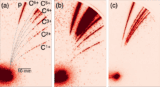
Graphene target boosts laser-driven ion acceleration
The researchers have already developed and implemented a device to help prevent damage to the cryostat caused by fast electrons and other particles emitted as a result of laser–target interactions. That device made the current study possible, the researchers say.
In the future, gases such as helium and argon could be used to produce other ion beams.
“We are preparing a new set of experiments where we want to apply the gained knowledge to, for example, further understand and optimize the acceleration mechanism as well as improve the stability of our acceleration process,” Rehwald says. “We think that potential applications of laser-driven proton accelerators will benefit from our research. For example, this could be of interest for new methods of radiation therapy in the future.”