
Fully contact-free laser ultrasound (LUS) imaging has been demonstrated in humans by researchers at Massachusetts Institute of Technology (MIT), in collaboration with MIT Lincoln Laboratory. Xiang Zhang and colleagues used an infrared laser to generate sound waves at the tissue surface of volunteers’ forearms. A second beam detected the propagating sound waves by measuring how the subjects’ skin vibrated in response. The technique could be especially useful for imaging where physical contact is not tolerated, such as over wounds and on other sensitive areas (Light Sci. Appl. 10.1038/s41377-019-0229-8).
In conventional ultrasound imaging, an array of transducers is pressed against the skin directly or with a coupling gel to help transmit the acoustic waves into the tissue. The method is inexpensive, convenient and produces images in real time, but it has some disadvantages.
One significant limitation is the pressure that is typically required to maintain acoustic contact between the device and the target tissue. This limitation is exacerbated for contact-sensitive applications where such pressure would be too painful, such as for burn victims or trauma patients.
Another problem is the low degree of reproducibility, due to the fact that the operator usually defines the image orientation and field-of-view by manipulating the transducers manually. This means that patient images acquired at different times are difficult to compare, and treatment or disease progression cannot easily be tracked.
A variation on the technique – ultrasound tomography – addresses the issue of reproducibility, but the solution comes at the expense of convenience, as it involves part of the patient being immersed in a water tank. The problem of physical contact, meanwhile, is partly dealt with by photoacoustic imaging. In this method, the ultrasound pulses are generated within the tissue remotely by a laser, but the reflected signal is detected using conventional transducers on the skin.
LUS could tackle these problems simultaneously by combining a new approach to photoacoustic generation with an optical interferometer, allowing it to create and measure ultrasound waves from a distance. Conventional photoacoustic imaging cannot image deeply since the light is strongly attenuated in the tissue. Rather than seeking a way to increase the laser’s penetration, however, in their new approach Zhang and colleagues turned this bug into a feature.
“We actually rely on this high absorption to efficiently generate an acoustic source at the tissue surface, meaning we can convert the maximum amount of light into acoustic energy while maintaining human safety,” explains Zhang. “This allows us to image deeper than typical photoacoustics since we don’t rely on light to travel through the tissue; only acoustic waves instead.”
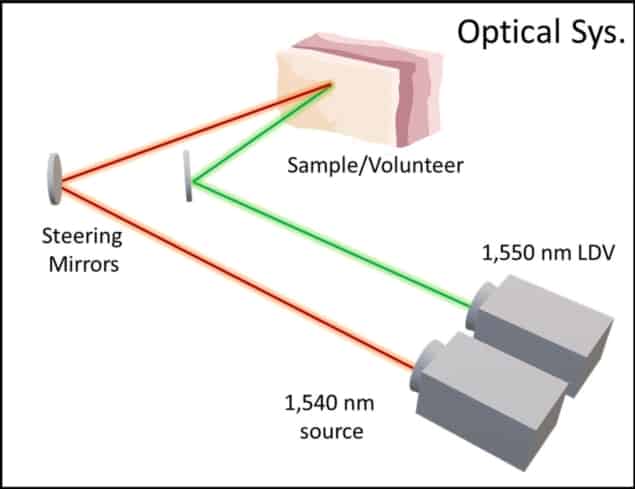
The team found that they achieved the ideal balance of optical absorption, acoustic power and patient safety using 2 mm-wide, nanosecond laser pulses at wavelengths near 1500 nm. This setup approximates a disk-shaped transducer just beneath the tissue surface, producing a 60° ultrasound beam at 1.5 MHz – towards the lower end of the frequency range typically used for ultrasound imaging.
The researchers tested their LUS technique using a gelatin phantom, ex vivo pig tissue and four human subjects, comparing the results to those from a standard ultrasound imager. While LUS could not match the image quality provided by the conventional approach, it still successfully picked out the same soft- and hard-tissue features.
One aspect in which LUS is currently lacking is its inability to deliver results in real time, as images must be reconstructed from sequential single-point measurements. In this respect, Zhang expects the development of the technique to mirror that of conventional ultrasound imaging.
“Looking back historically, medical ultrasound began by sequentially moving a single transducer to form an image – similar to moving a single laser spot in LUS – and eventually scaled toward arrays of hundreds or even thousands of transducers in medical probes today. I believe a similar path is ahead for LUS,” says Zhang.
Progress along this path should be accelerated by a fortunate coincidence: as well as being ideal for LUS, 1500 nm is the wavelength favoured by the telecommunications industry, meaning that both new and mature optical technologies are readily available for translation. Even in its current state of development, however, the technique could find immediate applications where high-quality images are not strictly required, Zhang suggests.
“For now, LUS could be useful in binary measurements where features don’t necessarily need to be resolved at a high resolution; rather, a yes/no measurement is sufficient, possibly for detection of internal bleeding or fractures in painful areas,” he tells Physics World.
Physics World’s Laser at 60 coverage is supported by HÜBNER Photonics, a leading supplier of high performance laser products which meet the ever increasing opportunities for lasers in science and industry. Visit hubner-photonics.com to find out more.