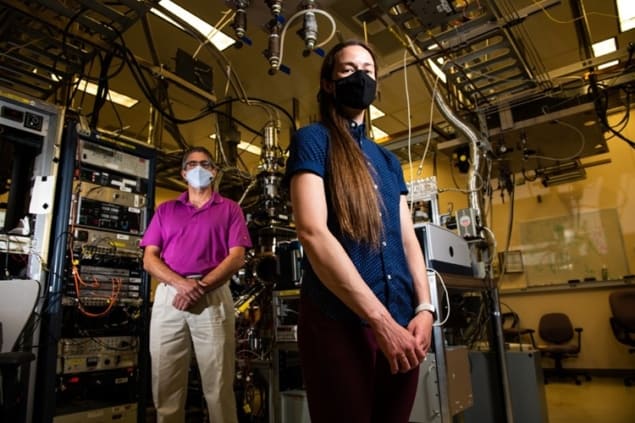
In an experimental first, scientists in the US have studied the dynamics of liquid water at temperatures below 230 K. Greg Kimmel and Loni Kringle of the Pacific Northwest National Laboratory in Richland, Washington used ultrafast laser pulses to “stop and start” the evolution of supercooled water in the nanoseconds before it froze, performing measurements in a temperature region that has been inaccessible to previous experiments. A paper describing their results is published in Proceedings of the National Academy of Sciences and suggests that the unusual properties of water might be attributed to the exchange of molecules between two coexisting liquid phases.
Water has more than 60 unusual properties that differentiate it from other liquids, including high heat capacity and a density that decreases upon freezing. There is evidence that these anomalies originate in the supercooled region, but despite decades of research, this remains unproven. Experiments on supercooled water are made almost impossible by a region between 160 and 230 K, which water researchers call “no man’s land”, where water crystallizes almost instantly.
Bridging the gap between theory and experiment
Kimmel has been studying supercooled water for more than two decades. Last year, Kimmel’s group showed, using an ultrafast laser heating technique, that water in the 160–230 K region always forms an equilibrium liquid before it crystallizes and that this liquid is a mixture of two structures, one high density and one low density.
Theorists have long predicted these two phases, but, as Kringle describes: “There is growing consensus that the anomalies of water, that are observable above 0 °C but become more pronounced upon supercooling, are related to the presence of these two structures but so little is known experimentally.”
The laser heating technique is fast enough that dynamics can be resolved as well as structure. As a supercooled water molecule moves through the liquid, it switches between high- and low-density motifs and the researchers wanted to know how this would affect the relaxation of the fluid.
Kringle and Kimmel entered this mysterious temperature region from below, heating amorphous (non-crystalline) ice at 70 K with laser pulses at rates of billions of degrees per second. The laser melted the water, but the heat dissipated after a few nanoseconds, cooling it so rapidly that the liquid structure was “locked in”. The fraction of low- and high-density water present after each laser pulse was measured with infrared spectroscopy, building up a series of “snapshots” of the liquid as it reached equilibrium.
Different densities, different dynamics
Like its liquid counterpart, amorphous ice can be high or low density. The researchers found that supercooled water relaxed to equilibrium more slowly if it started as low-density ice, though the final structures were similar. For both high- and low-density ice, they also found regions where the relaxation profile was a stretched exponential, which indicates molecules moving with lots of different speeds.
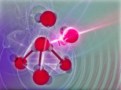
Supercooled water is stable in two different forms
Whether a water molecule can switch between structures on its way to equilibrium depends on how easily it can navigate the potential energy landscape. The researchers developed a model of exchange between potential wells where they took the fractions of high- and low-density water at each stage of the experiments and calculated the switching rates that would keep the system at chemical equilibrium. They found that this model fitted the experimental data surprisingly well; reproducing the stretched exponential and predicting that a smaller number of deeper minima makes it more difficult for low density water to structurally evolve.
A new perspective on supercooled water
For their conclusions to be valid, the researchers need to show that the potential energy landscape of amorphous ice is equivalent to its liquid counterpart. New research on supercooled water is always contentious, but Kringle described her surprise at how well the potential energy landscape model fitted their data, saying “While it’s not perfect, it does provide a starting point for understanding how the transition between two structural motifs results in stretched exponential relaxation.” Certainty is hard to come by below 0 °C, but this research suggests a link between water’s dynamics and its unusual structure.