
An Israeli-led collaboration has used atom-chip technology to build a magnet-based interferometer. The collaboration’s research, which combines aspects of two famous physics experiments from the past, reveals that the division of particle spins into two populations – as observed by Otto Stern and Walther Gerlach a century ago – involves pure quantum states. In the future, the new interferometer could be used to investigate quantum gravity as well as theories about a possible fifth force of nature.
Physicists have been discussing the possibility of magnet-based interferometry ever since Stern and Gerlach carried out their pioneering work on quantized angular momentum in the early 1920s. Their so-called Stern-Gerlach experiment used a magnetic field gradient to vertically deflect a beam of silver atoms from a straight path. A screen placed behind the magnet revealed that the atoms arrived at two distinct locations rather than forming a continuous line as predicted in classical physics.
This result provided convincing evidence that the silver atoms possessed an intrinsic and quantized angular momentum (now termed “spin up” and “spin down”). However, it did not prove that the atoms were in pure quantum states and therefore able to follow both trajectories simultaneously. As Ron Folman of Israel’s Ben-Gurion University of the Negev points out, individual atoms could have followed either one trajectory or the other, rather than both at the same time.
Interferometry advantage
To show that massive particles can exist in a quantum superposition, researchers have instead turned to interferometry. The goal here is to position each particle, or matter wave, in two distinct locations simultaneously, then bring the two wave packets back together and measure their interference pattern.
Until now, most interferometers developed for this purpose have interfered matter waves using a mixture of optical and physical gratings. Such devices have yielded interference fringes from molecules containing up to several thousand atoms, but extending the technique to much larger objects could prove tricky – in part because of the need to make gratings with ever narrower line spacings.

One promising alternative is to, in effect, combine the Stern-Gerlach experiment with the electron double-slit experiment by using magnetic fields not only to separate out the distinct wave packets, but also to bring them back together and interfere them. The presence of interference fringes would then prove there is a definite phase relation between the two wave packets, which is the hallmark of pure quantum states – just as an interference pattern appears in the double-slit experiment even when electrons pass through the slits one at a time.
Building such an interferometer has long been considered extremely challenging, given that both the position and momentum of the separated wave packets need to be restored with very high accuracy for fringes to form. But recent advances in atom chips have changed the outlook. These tiny electric circuits trap atoms, ions or molecules just above their surface, and make it possible to produce strong magnetic field gradients much more accurately than is possible using macroscopic coils.
A full loop
Folman and colleagues released individual atoms of rubidium-87 from a magnetic trap beneath an atom chip and used a radio-frequency pulse to place them in a superposition of two spin states. By sending pulses of current through the chip, they then exposed the free-falling atoms to four magnetic gradients in quick succession – splitting each atom’s wave packets along two paths, halting the wave packets’ relative movement, bringing them back together and finally halting them again.
As Folman explains, the free-falling configuration ensured the cleanest possible environment for the experiment. “The four operations are very delicate as the final two are supposed to exactly undo the action of the first two,” he says. “And if you want to perfectly close the loop, you don’t want any other fields – from the trap – to get in your way.”
In a previous study, the researchers observed interference using a simpler set-up known as a half-loop Stern-Gerlach interferometer, in which they separated the wave packets and then allowed them to expand over time until they overlapped. In contrast, the new “full-loop” interferometer actively brings the wave packets back together, and detects interference through variations in spin population rather than a spatial fringe pattern. The researchers say that this more complex technique should make it possible to observe the interference more quickly, and without the need for high-resolution imaging.
Towards a super-sized superposition
In a paper published in Science Advances, Folman and colleagues (including theorists in Germany, the Netherlands and the UK) describe how the complete interferometer might be used to study the superposition of much larger objects. In particular, they lay out the challenges involved in interfering the wave packets of a nanoscale piece of diamond containing about a million carbon atoms and a single nitrogen-vacancy spin embedded within them.
One especially difficult aspect of this problem, they write, will be to recombine the wave packets in a spot smaller than the coherence length of the nano-diamond “particle”. Although new cooling techniques could up the nano-diamond’s coherence length to about 0.1 nm, the overlap accuracy of their experiment was only 100nm. Nevertheless, they are optimistic that they can improve the accuracy by the necessary three orders of magnitude by driving the atom chip with more precise current pulses.
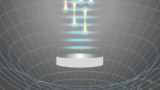
Trapped interferometer makes a compact gravity probe
If they can do that – while also minimizing environmental decoherence from scattered light, blackbody radiation or the magnetic fields – Folman says their device “could probe quantum mechanics and gravity in new regimes”. For example, detecting entanglement between two such devices placed side-by-side could put theories of quantum gravity to the test. Measuring gravitational interactions at short range might also make it possible to test for a putative fifth force. What’s more, they add, interferometers with large masses covering wide areas might be sensitive to new types of gravitational waves.
Gavin Morley of Warwick University in the UK, who was not involved with the latest research, is also upbeat. He argues that the new interferometer is “clearly ahead of competing technologies” when it comes to possible tests of quantum gravity. However, he cautions that the necessary enhancement of gravitational interactions and shielding of the nitrogen-vacancy spin will require nano particles with several billion, rather than million, atoms. “Smaller diamonds could test objective collapse theories and short-range gravity,” he adds.
- This article was amended on 8 June 2021 to include photos of the atom chip used in the experiment and a plaque commemorating the work of Stern and Gerlach.