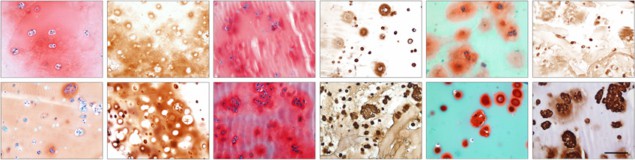
One of the challenges in using 3D printing to biofabricate cell-laden constructs for tissue repair and organ regeneration is ink optimization. Part of the puzzle is producing a printable blend, but that’s not the only consideration. It’s important to determine compositions that maximize the ability of cells to flourish and generate functional tissue.
“Here, factors such as the stiffness, composition and degradation rate of the ink play key roles in providing an appropriate niche to direct stem-cell fate,” explains Daniel Kelly, director of the Trinity Centre for Bioengineering, Ireland. “Highlights so far involve developing inks that can support vascular networks and engineer spatially complex tissues such as the osteochondral unit.”
Reporting their results in the journal Biofabrication, Kelly and his team have been comparing the performance of hydrogel bioinks by examining the printability of different blends and their capacity to support cartilage development.
Hydrogels (water-swollen polymers) have proven to be a useful addition to the mix. Softer versions of the material – with less cross-linking – favour the differentiation of cells, but construct developers also have to think about the mechanical integrity of their designs.
“This structural stability, often referred to as shape fidelity, is tightly related to the rheological properties of the bioink,” says Jos Malda, an expert in 3D bioprinting based at Utrecht University in the Netherlands. “An ideal bioink should exhibit shear-thinning behaviour – flowing as a low-viscosity fluid when extruded and behaving as a stable gel after printing.”
Malda points out that this transition should be as quick as possible to maintain the imposed shape, though the materials considerations don’t stop there. The target location of the biofabricated structure also adds to the list of design criteria and has led researchers to pursue a variety of solutions.
“The toughest applications revolve around implantation in mechanically challenging environments, which include bone, cartilage and tendons,” adds Malda. “To overcome such limitations we have been designing strategies to co-print bioinks with reinforcing thermoplastic polymers, hydrogels and microfibrous meshes.”
Fortunately, when it comes to screening potential bioinks there are some useful early indicators that can help to speed up the materials selection, particularly for characteristics such as printabilty and shape fidelity.

3D bioprinting smoothes path towards cartilage repair
“Tests based on yield stress and viscosity measurements, coupled with observations of how printed bioink filaments deform due to gravity or surface-tension effects, have been proven as simple but effective approaches to evaluate bioink shape fidelity,” says Malda.
Writing up their findings in the journal Biofabrication, Malda and his colleagues highlight yield stress as a key factor in determining the bioprintability of hydrogels based on gelatin-methacryloyl and gellan gum for cartilage repair.
Adding to the appeal of 3D printed scaffolds are other developments such as being able to tune the release profile of the various active elements contained in the structure. “Increasingly, we are also designing bioinks to act as delivery systems to temporally control the release of genes, growth factors and other regulatory cues to cells within printed constructs,” Kelly reveals.
- This article forms part of a series of reports reviewing progress on high-impact research originally published in the IOP Publishing journal Biofabrication.