
A matter-wave interferometer has measured the effect of gravity on individual atoms at the highest precision to date. That is according to its creators in the US and Italy, who built their instrument by combining matter-wave interferometry with the spatial control of optical lattices. The research was led by Cris Panda at the University of California, Berkeley and puts new constraints on some theories of dark energy involving “chameleon particles”.
Matter-wave interferometry is a powerful technique for probing fundamental physics. It takes advantage of wave–particle duality in quantum physics, which says that atoms behave as waves as well as particles.
“Lasers are used to split each atom in a quantum spatial superposition, such that each atom is effectively in two places at once,” Panda explains. “The two parts are then recombined and interfere either constructively, if the two parts are in-phase, or destructively, if they are out of phase.”
Search for new physics
When existing in two places, the phase of each component of the matter wave can be affected differently by external forces such as gravity. As a result, matter-wave interferometry can be used to make extremely precise measurement of the nature of these forces. This means that it can be used to search for deviations from the Standard Model of particle physics and Einstein’s general theory of relativity.
One fruitful area of investigation involves probing the gravitational force by placing a matter-wave interferometer next to a large mass. Atoms are split between locations at two different distances from the mass, allowing a comparison of the gravitational attraction between the atom and mass at two different places.
A shortcoming of such experiments, however, is that the atoms quickly fall out of place under Earth’s gravity, so making measurements longer than a few tens of milliseconds is difficult – limiting accuracy.
Atoms on hold
In 2019, the Berkeley team showed that optical lattices offer a solution by using lasers to hold atoms in position in Earth’s gravitational field. Earlier this year Panda and colleagues managed to hold atoms for 70 s in this way. Now, they have integrated a tungsten mass into their instrument.
Their latest experiment began with a gas of neutral caesium atoms that was cooled to near absolute zero in a magneto-optical trap. Some of the atoms were then transferred to a vertical optical lattice located just below a cylindrical tungsten mass that is about 25 mm in diameter and height (see figure).
A laser pulse was used to put the atoms into a superposition of two different micron-scale distances below the mass. There, they were held until a second pulse combined the superposition so that interference could be observed.
Information accumulation
“During the hold, the part of the atom in each location accumulates information about the local fields, particularly gravity, which can be read out at the end of the interferometer,” Panda explains. “The hold time can be many seconds and up to one minute, much longer than possible when atoms are falling under Earth’s gravity, which makes this device exceedingly sensitive.”
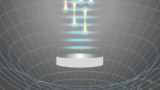
Trapped interferometer makes a compact gravity probe
While the experiment did not reveal any deviations from Newton’s law of universal gravitation, it did allow the team to put new constraints on some theories of dark energy – which is a hypothetical form of energy that is invoked to explain the universe’s accelerating rate of expansion. Specifically, the team put limits on the possible existence of “chameleon particles”, which are dark energy candidates that couple to normal matter via gravity.
The team is also confident that their technique could have exciting implications for a wide range of research. “Our interferometer opens the way for further applications, such as searches for new theories of physics through precise measurements of fundamental constants,” Panda says. “It could also enable compact and practical quantum sensors: such as gravimeters, gyroscopes, gradiometers, or inertial sensors.”
The research is described in Nature.