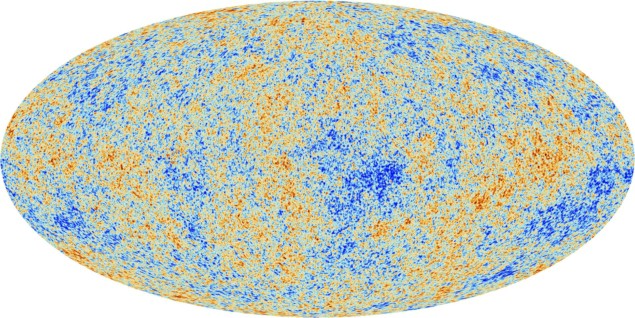
A theory of quantum gravity that describes the universe as beginning in a “Big Bounce” rather than a Big Bang has succeeded in explaining several anomalies in the cosmic microwave background (CMB) radiation.
Loop quantum gravity (LQG) is an alternative to string theory, and describes space itself as being quantized at the smallest scales, known as the Planck length, about 10–35 m. According to LQG, space cannot be crushed down any smaller than this, and the application of LQG to the broader Universe is known as loop quantum cosmology (LQC).
In standard Big Bang cosmology, were we to run the history of the universe backwards so that it collapses rather than expands, the universe would contract into an unknowable singularity. However, in LQC, the collapsing universe would stop collapsing at the Planck length, and then rebound. This suggests that if LQC is correct, there was no Big Bang singularity, but a Big Bounce resulting from the collapse of a previous universe.
Anomalous features
Now, new research by a team led by Abhay Ashtekar at Pennsylvania State University, has found that LQC can explain several anomalies in the CMB that have eluded explanation by other theories. The research is described in Physical Review Letters and the team includes Penn State’s Brajesh Gupt and Donghui Jeong, as well as Vijayakumar Sreenath of the National Institute of Technology Karnataka in India.
The work addresses two anomalies. One relates to the CMB’s power spectrum, which plots the tiny temperature variations in the CMB against their angular size. The other anomaly studied related to lensing amplitude, which is the degree by which the CMB light has been gravitationally lensed as it has travelled through the universe. Lensing is a result of the distribution and density of matter it passes through, which in turn is related to quantum fluctuations that rippled through the very early universe, prior to inflation.
If LQC is correct, then the Big Bounce should have influenced the properties of the CMB. In particular, LQC describes how, at the moment of the Big Bounce, the curvature of space-time was greater than at any other point in cosmic history.
“Loop quantum cosmology predicts a specific value for the curvature at the bounce,” Ashtekar tells Physics World. “This value is critical to get what we are seeing, namely that there are certain modifications of inflation, precisely at these large angular scales, that come from the specific nature of loop quantum cosmology.”
Imprint of the Big Bounce
The large curvature of space at the Big Bounce imprints specific fluctuations in the CMB that are of a wavelength far greater than the size of the visible universe, and so we cannot directly detect them. However, they also correlate with smaller wavelength modes that have a discernible effect on the CMB, in the form of the apparent anomalies that the Big Bang model cannot adequately explain.
There are six fundamental parameters that determine what we see when we look back at the CMB. Two of these parameters are primordial, relating to the end of inflation, and their values influence the power spectrum of the CMB. Two more relate to the time between the end of inflation, when the universe was about 10–32 s old, and the moment that the CMB light was emitted, some 379,000 years later. The final two parameters describe what happens between the time the CMB was emitted, and now. Although the Standard Model of Big Bang cosmology is able to determine values for these parameters, LQC modifies their values in such a way that the anomalies come naturally tumbling out of the data as products of the imprint of that extreme curvature at the Big Bounce.
“It’s pretty amazing that with these six parameters, cosmologists are able to predict what we see today,” says Ashtekar.
A third anomaly is a hemispherical one – the two hemispheres of the CMB have different average energies. Work by Ivan Agullo, of Louisiana State University, has already been able to address this anomaly in similar fashion, within the constraints of LQC. Agullo describes the work of Ashtekar’s group as “fantastic,” adding that “It proves that physical processes occurring in the remote past, before the inflationary epoch, can leave observable imprints in the sky.”
A fourth anomaly – the tension between measurements of the Hubble constant depending on whether you calculate it based on the CMB or via more local standard candles such as Type Ia supernovae – still awaits an explanation. However, Ashtekar points out that work by Alejandro Perez of Aix–Marseille University in France is taking the first steps at using LQC to solve this.