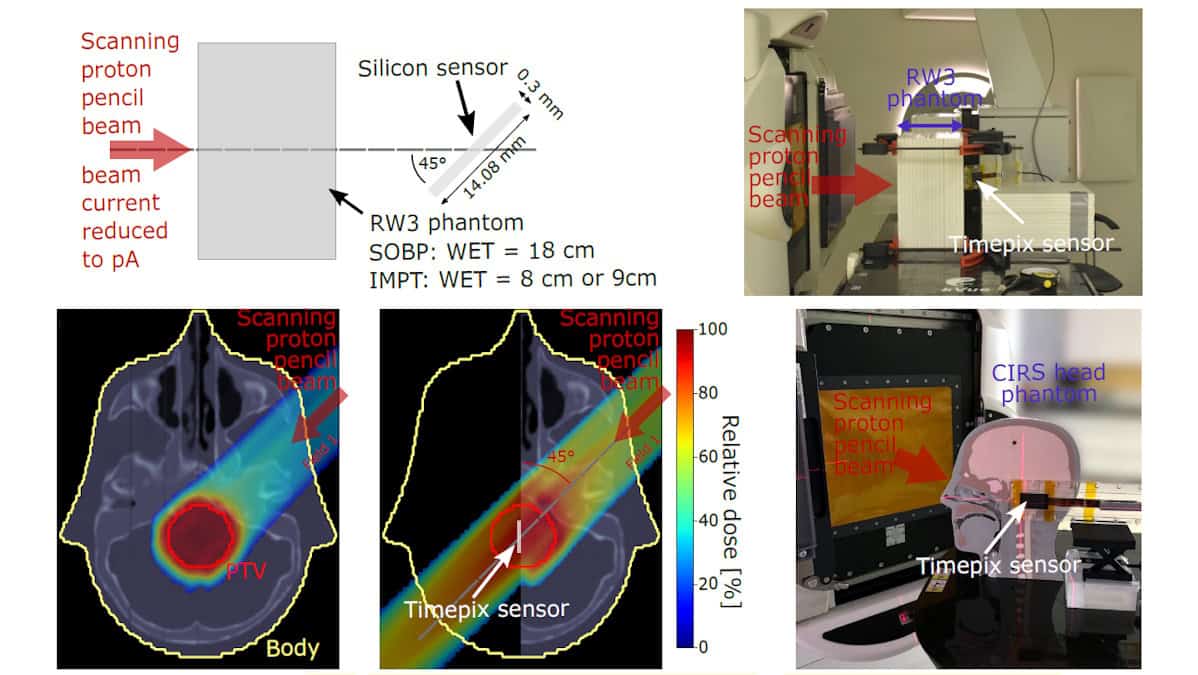
Proton therapy is a highly effective and conformal cancer treatment. Proton beams deposit most of their energy at a specific depth – the Bragg peak – and then stop, enabling proton treatments to destroy tumour cells while sparing surrounding normal tissue. To further optimize the clinical treatment planning process, there’s recently been increased interest in considering the radiation quality, quantified by the proton linear energy transfer (LET).
LET – defined as the mean energy deposited by a charged particle over a given distance – increases towards the end of the proton range. Incorporating LET as an optimization parameter could better exploit the radiobiological properties of protons, by reducing LET in healthy tissue, while maintaining or increasing it within the target volume. This approach, however, requires a method for experimental verification of proton LET distributions and patient-specific quality assurance in terms of proton LET.
To meet this need, researchers at the Institute of Nuclear Physics, Polish Academy of Sciences have used the miniaturized semiconductor pixel detector Timepix3 to perform LET characterization of intensity-modulated proton therapy (IMPT) plans in homogeneous and heterogeneous phantoms. They report their findings in Physics in Medicine & Biology.
Experimental validation
First author Paulina Stasica-Dudek and colleagues performed a series of experiments in a gantry treatment room at the Cyclotron Centre Bronowice (CCB), a proton therapy facility equipped with a proton cyclotron accelerator and pencil-beam scanning system that provides IMPT for up to 50 cancer patients per day.
The MiniPIX Timepix3 is a radiation imaging pixel detector based on the Timepix3 chip developed at CERN within the Medipix collaboration (provided commercially by Advacam). It provides quasi-continuous single particle tracking, allowing particle type recognition and spectral information in a wide range of radiation environments.
For this study, the team used a Timepix3 detector with a 300 µm-thick silicon sensor operated as a miniaturized online radiation camera. To overcome the problem of detector saturation in the relatively high clinical beam currents, the team developed a pencil-beam scanning method with the beam current reduced to the picoampere (pA) level.
The researchers used Timepix3 to measure the deposited energy and LET spectra for spread-out Bragg peak (SOBP) and IMPT plans delivered to a homogeneous water-equivalent slab phantom, with each plan energy layer irradiated and measured separately. They also performed measurements on an IMPT plan delivered to a heterogeneous head phantom. For each scenario, they used a Monte Carlo (MC) code to simulate the corresponding spectra of deposited energy and LET for comparison.
The team first performed a series of experiments using a homogeneous phantom irradiated with various fields, mimicking patient-specific quality assurance procedures. The measured and simulated dose-averaged LET (LETd) and LET spectra agreed to within a few percent, demonstrating proper calibration of the measurement methodology.
The researchers also performed an end-to-end test in a heterogeneous CIRS head phantom, delivering a single field of an IMPT plan to a central 4 cm-diameter target volume in 13 energy layers (96.57–140.31 MeV) and 315 spots.
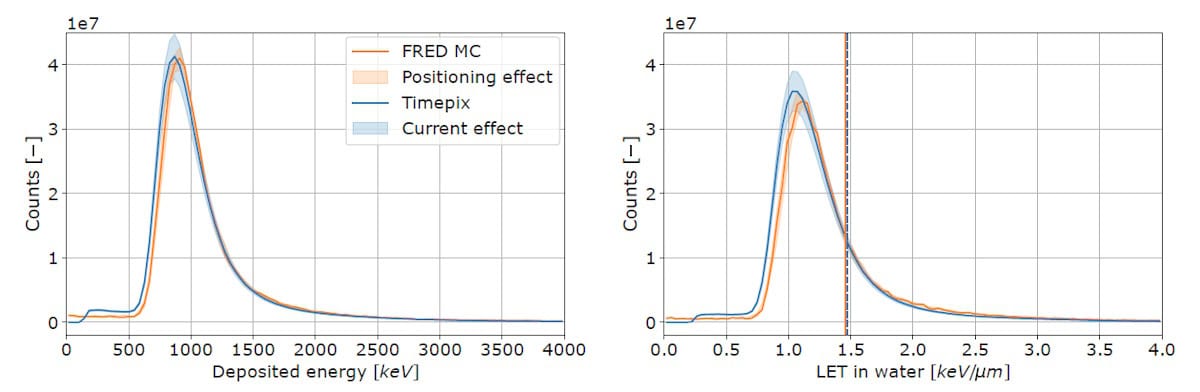
For head phantom measurements, the peak positions for deposited energy and LET spectra obtained based on experiment and simulation agreed within the error bars, with LETd values of about 1.47 and 1.46 keV/µm, respectively. The mean LETd values derived from MC simulation and measurement differed on average by 5.1% for individual energy layers.
Clinical translation
The researchers report that implementing the proposed LET measurement scheme using Timepix3 in a clinical setting requires irradiating IMPT plans with a reduced beam current (at the pA level). While they successfully conducted LET measurements at low beam currents in the accelerator’s research mode, pencil-beam scanning at pA-level currents is not currently available in the commercial clinical or quality assurance modes. Therefore, they note that translating the proposed approach into clinical practice would require vendors to upgrade the beam delivery system to enable beam monitoring at low beam currents.
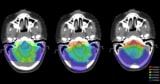
Proton therapy planning: how to minimize LET in organs-at-risk
“The presented results demonstrate the feasibility of the Timepix3 detector to validate LET computations in IMPT fields and perform patient-specific quality assurance in terms of LET. This will support the implementation of LET in treatment planning, which will ultimately increase the effectiveness of the treatment,” Stasica-Dudek and colleagues write. “Given the compact design and commercial availability of the Timepix3 detector, it holds promise for broad application across proton therapy centres.”