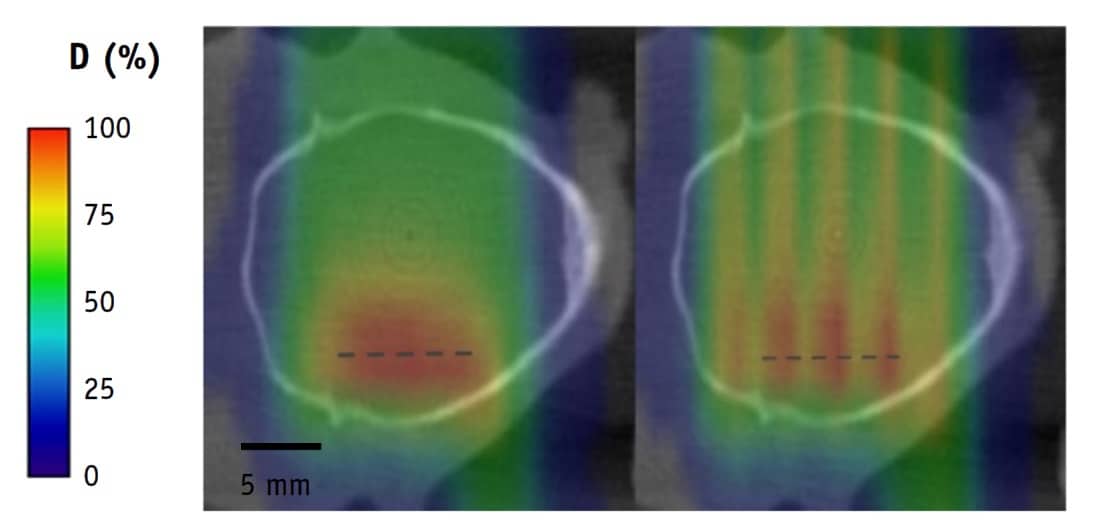
Advances in the technologies used to deliver radiation therapy have enabled improved tumour conformity along with reduced dose to nearby organs-at-risk. However, the dose tolerance of normal tissues remains a limiting factor in radiotherapy dose delivery. This leads to difficulties in treating radioresistant tumours, for example, or cancers near highly sensitive structures, such as paediatric brain tumours.
One option could be to employ new modes of radiation dose deposition, for example, delivering spatially fractionated dose using an array of submillimetre-sized radiation beams. At the recent ESTRO 38 meeting in Milan, Yolanda Prezado from CNRS described the rationale for this novel approach.
In its most extreme, she explained, microbeam radiotherapy uses beams of around 50 µm in width spaced by a few hundred microns. This creates an inhomogeneous dose distribution with alternating high peak doses and low valley doses. When using such radiation beams, normal tissue can withstand doses as high as 300 Gy in one fraction. However, such small field sizes and high dose rates can only be created by synchrotron sources.
“This concept is fascinating, but clinical trials are very difficult because these very high dose rates are confined to synchrotrons,” said Prezado. “Instead, it may be a good compromise to use minibeam radiotherapy.”
Minibeams have a width of between 500 and 700 micron, and can be produced by lower-cost equipment other than synchrotrons, allowing a more widespread implementation. Prezado described a proof-of-concept study demonstrating that such minibeams still provide normal tissue sparing in glioma-bearing rats compared with conventional radiotherapy. As well as eliminating normal tissue damage, the minibeams also increased tumour control, she noted.
Moving forward, Prezado introduced a newer concept: proton minibeam radiotherapy (pMBRT). In a first evaluation of this approach, Prezado and colleagues compared whole-brain irradiation of glioma-bearing rats with either standard proton therapy or pMBRT, both using a prescription of 25 Gy in one fraction (Int. J. Radiat. Oncol. Biol. Phys. 10.1016/j.ijrobp.2019.01.080).
They found that the standard treatment caused skin damage and substantial brain damage in the animals. Treatment with pMBRT, however, which delivered a peak dose of 58 Gy, resulted in no skin damage and a net reduction in toxicity. The minibeam treatment also increased the animals’ long-term tumour-free survival, from 22% to 67%.
“This is among the best results ever obtained with radiotherapy alone, and with a reduction in neurotoxicity,” Prezado noted.
She suggested that pMBRT could provide a novel radiotherapy concept to solve an urgent medical need, namely the ability to increase the tolerance doses of normal tissues. This, in turn, could reduce the risk of complications in paediatric cancer therapy, enable dose escalation to treat radioresistant tumours, and even reduce costs by enabling hypofractionation and reducing targeting requirements. Preparations for the first clinical trials are starting, she told the delegates.
Another potential approach for the future could be to employ minibeams of heavy ions. Prezado explained that ion beams of 500 µm in width can be generated and that using a large beam spacing minimizes the contribution of high-LET fragments to the valley dose, generating an extremely high peak-to-valley dose ratio. She noted that a dosimetric study had shown that heavier beam fragments are dominant in the valleys only at the target position, which may help increase tumour control.
Prezado concluded by pointing out that the dose delivery method may impact the tumour’s biological response — and may actually be more important than the dose level itself. “I think that for clinical practice, it’s a good choice to fully exploit spatial fractionation,” she said.