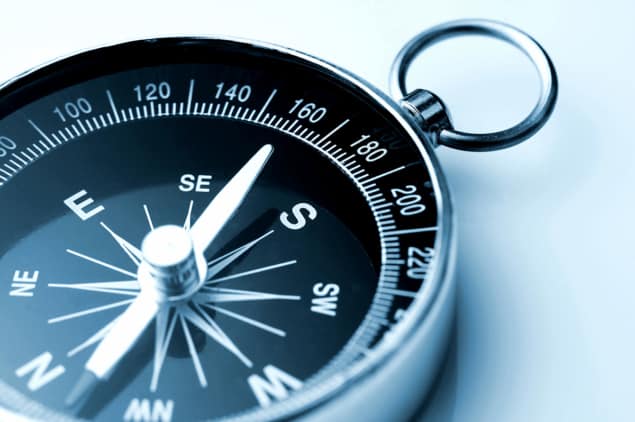
Scientists in China have devised what they describe as the molecular equivalent of a compass to measure the weak van der Waals interactions between atoms. They did so by using a new kind of electron microscopy to track the rotation of a single hydrocarbon molecule within a crystal. The scientists reckon their tiny sensor could provide new insights into molecular-scale processes such as catalysis and phase transitions.
Van der Waals forces arise from temporary fluctuations in the density of charges in neighbouring atoms or molecules. Although they are only effective over a limited distance, they are ubiquitous in nature and widely exploited in industry, playing key roles in fields from condensed matter physics to structural biology. However, measuring them directly usually requires sophisticated techniques best suited to the study of single atoms.
In the latest work, Fei Wei and Xiao Chen at Tsinghua University in China and colleagues turn to a snappily named technique called integrated differential phase contrast scanning transmission electron microscopy (iDPC-STEM). Like other forms of electron microscopy, iDPC-STEM exploits the short de Broglie wavelength of energetic electrons to create images at far higher resolution than is possible with light waves. However, it has a couple of important advantages over other types of electron microscopy.
Developed by researchers at Thermo Fisher Scientific in Eindhoven, Netherlands, (including current group members Eric Bosch and Ivan Lazić) it uses integrated image data, which yields a higher signal-to-noise ratio for a given flux of electrons and thus allows smaller electron doses to be used. In addition, its image contrast scales roughly linearly with atomic number Z, rather than with Z2, making it more suited to studying systems with both light and heavy elements.
Single molecule in a crystal
The system in the Tsinghua team’s experiment is very sensitive to electron beams, and is made from single molecules of para-xylene (containing eight carbon and ten oxygen atoms each) trapped within the voids of a zeolite crystal. (Zeolites are microporous minerals that occur naturally but are also produced industrially on a large scale.) The type of zeolite used in this research, known as ZSM-5, consists of rings of silicon and oxygen atoms that link up around large holes in a two-dimensional lattice sheet. When a few of these sheets are stacked on top of one another, the holes align, creating shallow channels through the structure. It was into these channels that Wei and colleagues placed their para-xylene molecules by mixing ZSM-5 powder and para-xylene liquid in a centrifuge.

To make their compass, Wei and colleagues took advantage of the fact that the perimeter of each hole consists of a ring of 10 silicon and 10 oxygen atoms interspersed at roughly equal intervals of 18 degrees. The idea was to use the para-xylene molecule inside each ring as a pointer. Because any shift in the molecule’s axis relative to the silicon and oxygen atoms (the “compass points”) would indicate changes in local van der Waals interactions, the researchers could determine the nature of these changes by imaging the molecule and observing the orientation of its long axis in the plane of the ring.
Tiny force changes
Wei and co-workers showed they could indeed use their compass to measure force changes in both space and time. They did this by comparing changes in the orientation of the para-xylene pointers, as seen in the images provided by iDPC-STEM, with variations in the shape of the (slightly elliptical) rings. They gauged these variations by using intensity measurements to establish the distance between pairs of atoms on opposite sides of the rings.
The researchers compared multiple rings and found that the pointers tend to line up along the major axis in each ellipse. They also found that the pointer in any given ring tends to move between compass points so that it stays aligned with the longest axis as the ring changes shape (as a result of increasing exposure to electrons).
To relate these responses to changes in van der Waals interactions, the researchers used first principles calculations to work out how the para-xylene molecules’ interaction energy ought to vary with ring geometry. They found that for each different ellipse, it was always energetically favourable for the molecule to line up along the longest axis – demonstrating, they say, that each molecule in its crystal void does indeed function as a “van der Waals compass”.
Challenges of interpretation
Chen argues that the work could have applications in optimizing zeolite-based catalysis – a process used, among other things, to convert alcohol into petrol. As she points out, ZSM-5 contains aluminium atoms, which can provide protons during the acid-base interactions vital to such catalysis.
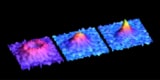
Single-molecule magnetometer images spin interactions
Other experts have responded with a mixture of enthusiasm and caution. Shigeki Kawai of the National Institute for Materials Science in Tsukuba, Japan, says that while electron microscopy has already been used to image single molecules, no-one has previously anchored a single molecule inside zeolite pores. This capability, he reckons, might allow two different molecules to be aligned or even reacted with one another.
Bart Kooi of the University of Groningen in the Netherlands, meanwhile, praises Wei and colleagues’ “nicely prepared samples and state-of-the-art imaging”. However, he adds that using two-dimensional images to represent three-dimensional structures complicates the process of calculating the interaction energy. He also questions the extent to which the imaging electrons – even in low doses – might still affect the atomic structures being studied.
The research is published in Nature.