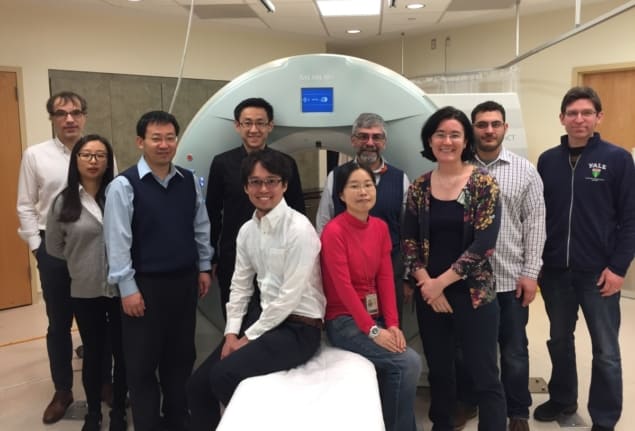
PET, an established imaging modality employed for both clinical and research applications, has the potential to perform in vivo radiotracer quantitation. Such absolute quantification directly measures the physiological parameter of interest, which may yield more clinical efficacy. This potential, however, may be compromised by body motion, which degrades image resolution and alters apparent tracer uptake.
To help realise the full potential of quantitative PET, researchers at Yale University have investigated the use of the Centroid of Distribution (COD) algorithm to detect body motion and perform event-by-event non-rigid motion correction during image reconstruction (Phys. Med. Biol. 10.1088/1361-6560/ab02c2).
For each list-mode event, the COD algorithm calculates the central coordinate of the time-of-flight (TOF) bin, a surrogate for the annihilation point. These coordinates are then averaged over a short time interval (1 s in this study) to generate a COD trace. The trace comprises three components: CODY (anterior–posterior) and CODZ (superior–inferior), which have been used previously to track respiratory motion, and CODX (lateral), which the authors propose will be the most sensitive to body motion.
The COD traces include both motion-free frames (MFFs) and abrupt changes that indicate movement. To estimate body motion, reconstructed MFF images are non-rigidly registered to a reference frame. The resulting displacement represents the motion vector field between the two MFFs. These motion vector fields, along with the list-mode data and CT attenuation map, are used in the NR-MOLAR algorithm, which performs event-by-event non-rigid body motion compensated (NR-BMC) reconstruction.
According to first author Yihuan Lu, COD-based motion detection would be easy to implement in the clinic. “The only information needed to calculate COD is the PET data itself, specifically detector location and the time-of-flight information of the event,” he explains. “There is absolutely no requirement on additional systems for motion detection. In addition, computation of the COD is ultrafast and can be computed in real time during the PET scan.”
Resolution recovery through motion correction
To validate their motion correction scheme, Lu and colleagues performed six consecutive 10-min PET scans of an anaesthetized monkey, 10 minutes after injection with 18F-FDG. Half way through each scan, they moved the monkey in different ways: lateral translation by 0.5 and 1 cm; rotation; reverse-rotation; and 2 cm superior–inferior translation. During the sixth scan, they moved the animal in four different ways. The CODX trace was the most sensitive to body motion, successfully detecting all nine movements.
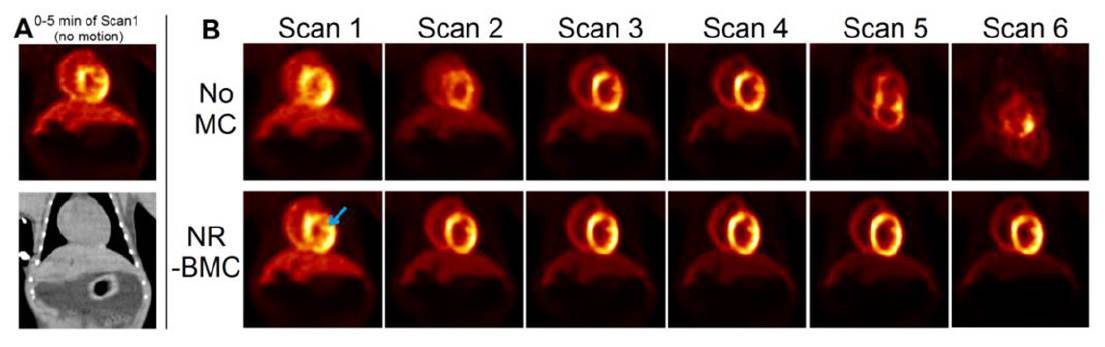
PET images reconstructed without motion correction exhibited significant resolution loss and blurring in cases with translation, and slight image quality degradation when the animal was rotated. Reconstruction using NR-BMC effectively recovered the resolution and contrast in all cases, with consistent spatial alignment among all scans after motion correction.
The team also retrospectively analysed four human studies and a dog enrolled in a cardiotoxicity study. In all cases, they compared their NR-BMC method with no motion correction, rigid and non-rigid post-reconstruction registration (R-PRR and NR-PRR) and rigid-body motion correction (R-BMC), which uses the COD algorithm but with rigid registration.
The studies included two patients imaged using 18F-FPDTBZ, which binds to pancreatic β-cells. In the first case, PET images reconstructed using no motion correction, R-PRR or R-BMC contained “two” pancreases, due to large body motion. NR-PRR substantially improved resolution and contrast, while NR-BMC outperformed all other methods for all organs.
In the second patient, who exhibited frequent rotational movements, NR-BMC again achieved the highest motion correction, particularly for organs with thin structures such as the pancreas and kidney.

The researchers also examined a patient with non-small cell lung cancer scanned using the hypoxia tracer 18F-FMISO. In this case, motion was mainly seen in the arm and NR-BMC effectively restored the bone–muscle boundary. In another example, an 18F-FDG study of a patient with lung nodules, R-BMC and NR-BMC outperformed the other methods in terms of resolution and contrast recovery.
Finally, they examined data from the dog, which was scanned with 18F-DHMT to image myocardial reactive oxygen species. Images without motion correction were blurred, in particular the wall of right ventricle myocardium, due to body motion. R-PRR and R-BMC slightly recovered myocardium resolution, while NR-BMC and NR-PRR more effectively restored resolution.
The authors conclude that the COD algorithm with event-by-event NR-BMC can effectively detect and correct motion in PET scans of both humans and animals, for multiple tracers, with superior performance to conventional post-reconstruction registration approaches.
“Given the initial success of the proposed COD approach in single-bed body motion detection and correction, we are now working on extending its application to whole-body PET, which is a necessary step to achieve its full potential in routine clinical practices,” Lu tells Physics World. “Meanwhile, we are working on simultaneous correction of multiple motion types, for example, respiratory and voluntary body motion.”