Researchers in the US have used an alternative form of magnetic resonance imaging (MRI) to visualize objects with a resolution of just 90 nm. The best conventional MRI microscopes, in contrast, typically have a resolution of about 3 µm. The researchers claim the technique, known as magnetic resonance force microscopy, could be used to map out the structure of nanometre-scale structures such as proteins and pharmaceutical drugs (Nature Nanotechnology doi:10.1038/nnano.2007.105)
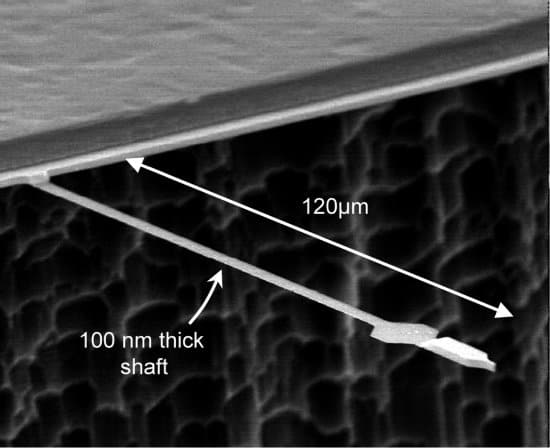
MRI is widely used in medicine, biology and materials science because it can make non-intrusive 3D images of materials. The technique involves placing a sample in a magnetic field and then transmitting a radio-frequency pulse through the sample via a metal coil. The radio waves drives the spin of some of the nuclei out of alignment with the magnetic field. Together, these nuclei create a bulk magnetic moment that induces a current in the coil and in turn provides information about the local chemical nature of the sample.
Conventional MRI is limited to a resolution of several micrometres, being restricted by how small the coils that generate and detect the radio waves can be made. Now, however, John Mamin and colleagues at IBM’s Almaden Research Center in California have found a way around this restriction by doing away with the detection coils and using a mechanical cantilever instead. Although their technique of magnetic resonance force microscopy (MRFM) is not new, the IBM team have been able to improve its resolution to just 90 nm.
The technique involves coating a sample onto the free end of a 120-µm long cantilever, which vibrates at its natural frequency. The sample — in this case a test object made from tiny rectangular blocks of calcium fluoride — lies just above a tiny magnetic tip made of a cobalt-iron alloy that generates an intense and highly-localized magnetic field.
A nearby coil generates regular radio pulses, which are tuned to reverse the alignment of the nuclear spins of fluorine-19 atoms in the sample. Thanks to the motion of the vibrating cantilever, the portion of the sample that is nearest to the magnetic tip moves back and forth though the highly-localized magnetic field. This results in a force being exerted on any fluorine atoms that happen to be near the tip. This force changes the resonant frequency and amplitude of oscillation of the cantilever, which can then be detected using fibre-optic interferometry.
The magnitude of these changes is proportional to number of fluorine atoms in the region of the magnetic field, allowing a 2D image of the calcium fluoride concentration to be made by scanning the tip across the sample. The researchers claim that they were able to makes images of as few as 1200 atoms in a sample comprising rectangular blocks of calcium fluoride that were 80 nm tall and 180 nm wide. The blocks varied in length from 165 nm and 295 nm and were arranged in a row, separated by distances as small as 50 nm. The microscope imaged all the features on the sample with the exception of the 50-nm gap – leading the researchers conclude that the resolution of the microscope is 90 nm (see figure “90 nm resolution”). This is equivalent to a detection volume of volume of just 650 zeptolitres, which is some 60,000 smaller than is possible with the best conventional MRI and at least 70,000 times smaller than with the best MRFM to date.
Although the new microscope represents a major improvement in MRI microscopy, the measurements had to be done in a vacuum chamber at temperatures as low as 600 mK in order to minimize noise in the cantilever. As a result, measurements cannot be made under ambient conditions, which precludes its use in the study of many of materials – particularly some biological samples.