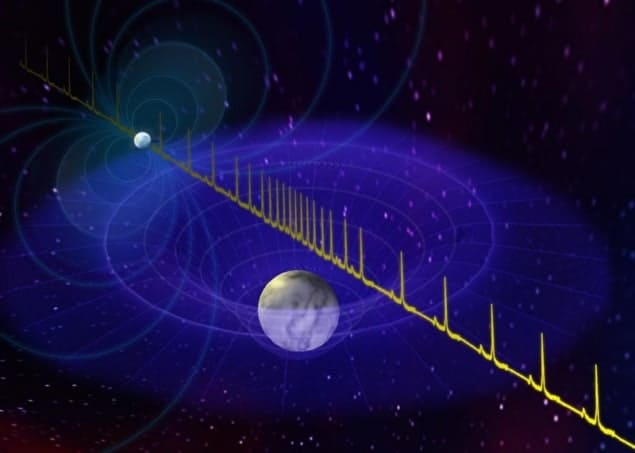
Using a technique that exploits general relativity, astronomers in the US and the Netherlands have identified a neutron star that has a mass nearly twice that of the Sun. The object is the most massive neutron star ever determined with reliable precision, and its existence, say the researchers, rules out much of the exotic matter hypothesized to occur inside these ultra-dense burnt out stars.
Neutron stars form when stars exhaust all of their nuclear fuel and implode under their own weight. The immense gravity forces protons and electrons together, leaving a ball made up largely of neutrons that has a density up to ten times that of atomic nuclei. Theorists have proposed that the huge pressure inside such an object could lead to a number of different forms of exotic matter. One of these is a material known as a Bose–Einstein condensate, in which particles act together as a single quantum entity. Alternatively, the neutrons might split to form a “soup” of free quarks and create what is known as a “quark star”.
The bigger the better
One way to discriminate between these different hypotheses is to look for the existence of very massive neutron stars. Exotic particles (if they existed) would repel each other less than neutrons do, and so would provide less resistance against gravitational collapse. This means that the mass value at which an exotic neutron star implodes to form a black hole would be lower than that of a conventional neutron star. In other words, an object of this composition could not exist above a certain mass.
Astronomers have previously identified neutron stars that might weigh as much as 2 solar masses, which is significantly greater than the 1.4–1.5 solar masses considered typical of neutron stars. However, such mass measurements have been imprecise. The new work, carried out by Paul Demorest of the National Radio Astronomy Observatory (NRAO) in Virginia and colleagues, takes advantage of an effect of general relativity known as the Shapiro delay, which is the delay experienced by a radio signal as it passes through the gravitational potential of a massive object.
The idea is to measure very precisely the arrival time of radio waves from a spinning neutron star known as a pulsar, which is in orbit around a centre of gravity shared by a companion star. The pulsar emits bursts of radio waves separated by a very well defined interval – generally a few thousandths of a second – and this interval will be delayed very slightly as the companion star passes between it and the Earth, owing to the companion star’s gravitational field. The exact shape of the curve that describes how this delay varies throughout the cycle of the binary system reveals the inclination of the orbital plane compared to the Earth’s line of sight, while the magnitude of the delay tells us the mass of the companion star. Combining these data with measurements of the orbital period and the pulsar line-of-sight speed yields a value for the pulsar mass.
A clean way to measure
Demorest and co-workers point out that this approach is a very “clean” way of measuring the pulsar mass because, unlike alternative methods, it relies on a single type of data. But the Shapiro delay is a very weak effect. The researchers were able to optimize their measurements by using a binary system, known as J1614-2230, in which the orbital plane is almost exactly edge-on as seen from the Earth and in which the companion star is comparatively massive – both characteristics that increase the magnitude of the delay. They observed the system over the course of one complete nine-day orbit in March this year using an instrument known as GUPPI on the NRAO’s Green Bank radio telescope. From these observations they calculated the pulsar to have a mass of 1.97 solar masses, with error bars of ±0.04 solar masses (Nature 467 1081).
This figure is significantly higher than the previous record for precisely measured neutron star mass – 1.67±0.01 solar masses. And it is, say the researchers, high enough to rule out a wide range of models positing the existence of exotic matter inside neutron stars. Feryal Ozel of the University of Arizona, lead author of a companion paper to be published in Astrophysical Journal Letters, says that among the exotic particles to get the chop are hyperons, kaon condensates and free quarks. She says that if quarks are to exist in the core of neutron stars then they must strongly interact with one another, as they do inside normal matter, in order to withstand the star’s huge gravitational field.
Frits Paerels of Columbia University in New York, who was not involved with the work, agrees. “This measurement really does not appear to leave a lot of wiggle room for models of neutron stars that involve exotic condensates,” he says. “It also starts to close in on the models based on quark matter. Indeed, it comes very close to ruling out quark stars.”