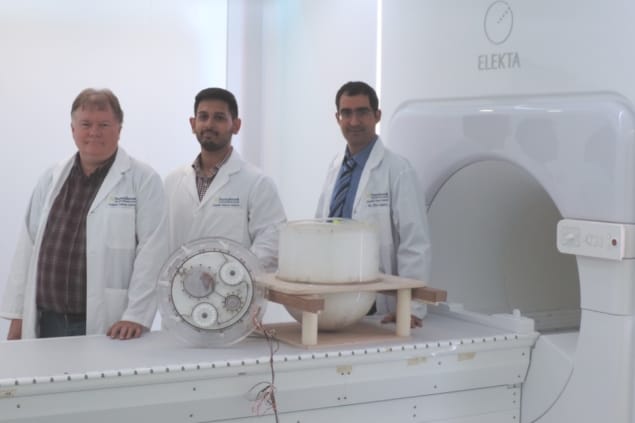
With the introduction and proliferation of MRI-guided radiotherapy systems, there’s a crucial need to develop MR-compatible strategies for absolute dosimetry and beam measurement. At the recent AAPM Annual Meeting, delegates heard about two novel approaches for MR-linac dosimetry.
Water calorimetry
Humza Nusrat from Ryerson University described the development of a portable water calorimeter for MR-Linac dosimetry. The work is being performed under the supervision of Arman Sarfehnia in the radiation treatment program of Odette Cancer Centre, Sunnybrook Health Sciences Centre. “Water calorimetry involves measuring the minute temperature rises that result from radiation exposure to water,” he explained.
Calorimetry is of particular interest as it provides a direct measurement of absolute absorbed dose and can be used as a primary standard. Radiation-induced temperature changes, however, are on the order of millikelvins and thus require highly sensitive detection devices. Another challenge is the “heat defect”, in which radiation-induced chemical changes in the water cause the temperature rise to differ from that expected for complete conversion of absorbed energy.
Nusrat and colleagues designed the water calorimeter using finite element method analysis to optimize thermal stability and performance. To address the heat defect problem, they enclosed the heat detectors (thermistors) within a glass vessel containing highly pure water saturated with hydrogen gas.
The calorimeter was constructed using MR-compatible plastic and ceramic components, and the portable water tank containing the glass vessel was operated at 4°C to minimize heat transfer. Thermal stability was also maintained using hydraulic stirrers and thermal insulators. “We have designed and built a water calorimeter that can operate in variety of radiotherapy settings,” said Nusrat.
The team used the device to perform calorimetry measurements on the Elekta MR-linac. The calorimeter was clearly visible in a 1.5 MR image, enabling its accurate positioning within the radiation field. They recorded initial measurements with the magnetic field switched off.
Following the calorimetry, the thermistors were removed from the glass vessel and replaced with a thimble ionization chamber to compare dosimetry results. The water calorimetry results agreed with the ion chamber dosimetry to within 2%, with the calorimeter recording a slightly higher dose.
Nusrat noted that improving the window insulation may improve this correspondence further. The next step in this project, he says, will be to repeat the calorimeter measurements with the magnetic field switched on.
Cherenkov checks
Presenting in the same conference session, Jacqueline Andreozzi of Dartmouth College discussed the use of Cherenkov imaging for beam measurements in MR-linacs.
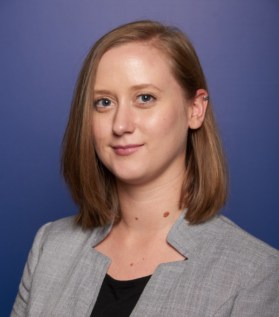
Cherenkov light is produced by the therapeutic photon beam as it passes through tissue, or water. Here, the Dartmouth researchers used an intensified CMOS sensor to measure Cherenkov emission in a water tank irradiated by an MR-linac. They employed a remote triggering device to synchronize image acquisition to the radiation pulses.
Andreozzi explained that, although most Cherenkov light is emitted in the blue spectrum, and the team’s sensor is more sensitive to red/near-infrared photons, they demonstrated in simulations that the number of detected Cherenkov photons is proportional to radiation dose.
The researchers irradiated the tank with 6 MV beams from the MR-linac, from the largest possible (24.07 x 27.20 cm) to the smallest (2 x 4.15 mm) field size. They used the Cherenkov images, which represent a 2D integration of the delivered dose, to create projection percentage depth-dose (PDD) maps. These were then compared to summed projection images of dose from the treatment planning system (TPS).
Andreozzi first described measurements on larger beams, with field sizes of 4.98 x 4.98, 9.96 x 9.96, 14.94 x 14.94 and 27.2 x 24.07 cm. For these beams, PDD measurements from Cherenkov projections matched those from the TPS, with an average error of 0.8%, for example, for the smallest of these beams.

In agreement with the team’s previous findings on a Co-60-based MRI-guided radiotherapy system, the results confirmed that real-time Cherenkov measurements can provide an accurate, quick check of beam output with no correction factors necessary.
Moving onto smaller field sizes, Andreozzi pointed out that despite delivering more dose (1000 MU per image, compared with 200 MU for the larger beams), Cherenkov intensities for small beams are a fraction of those for large beams. “Small beams present a challenge, because we are limited by the efficiency of collecting photons,” she explained.
Here, average errors were 3.7%, 0.31% and 0.44%, for field sizes of 2 x 4, 8 x 8 and 16 x 16 mm, respectively. Andreozzi noted that it may be possible to increase the signal-to-noise ratio by increasing the aperture size. Results should also be improved with the use of a new blue-sensitive camera. “The blue-sensitive camera provides a 150% average dose increase; this will translate nicely to small beams,” she said.
Andreozzi concluded that Cherenkov imaging provides a rapid, repeatable and MR-compatible beam measurement method for MR-linacs, with the potential to be expanded to small beam dosimetry. “We are studying the same technology to experimentally image dose variation due to the electron return effect in MR-linacs, as well as to develop a technique for rapid, quantitative alignment of the MRI and radiotherapy isocentres,” she tells Physics World.