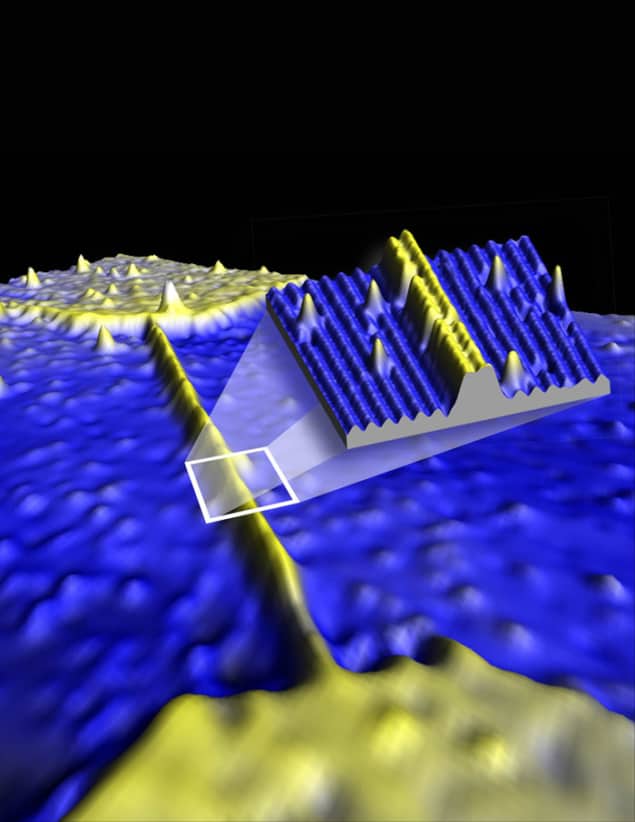
A new technique for embedding atomic-scale wires within crystals of silicon has revealed that Ohm’s law can hold true for wires just four atoms thick and one atom tall. The result comes as a surprise because conventional wisdom suggests that quantum effects should cause large deviations from Ohm’s law for such tiny wires. Paradoxically, the researchers hope the finding will aid the development of quantum computers.
As chipmakers pack increasing numbers of circuits onto silicon wafers, the size of transistors and other devices are nearing the atomic scale. Beyond the sheer technological challenges of making ever-smaller components, many physicists are concerned that the inherent fuzziness of quantum mechanics will soon render the familiar classical laws of electronics obsolete.
To investigate conduction on the atomic scale, Michelle Simmons, Bent Weber and colleagues at the University of New South Wales in Australia have developed a method of using phosphorus atoms to embed atomically thin conducting regions within a crystal of bulk silicon. Phosphorus has one more electron in its outer shell than silicon and if a silicon atom is replaced by a phosphorus atom (a process called n-doping), it donates a free electron to the crystal, thereby raising the conductivity of the doped region.
“Remarkable achievement”
In what condensed-matter physicist David Ferry of Arizona State University in the US describes as “a remarkable achievement”, Simmons’ team use the tip of a scanning probe microscope to create a channel in the silicon by removing layers of silicon atoms. The surface is then exposed to phosphorus gas, followed by the deposition of silicon atoms. The result is a chain of phosphorus atoms embedded inside a silicon crystal – effectively an atomic wire. The team found that the resistivity of these wires was constant right down to the atomic scale. This means that the resistance of such a wire is proportional to its length and inversely proportional to its area, just as you would expect from Ohm’s law.
Although Simmons says the techniques used to create the wires cannot currently be deployed in industrial processes, Ferry believes it is a valuable demonstration that, in principle, the miniaturization of classical electronics can continue for several years. “Firms such as Intel have been worried about making their devices so small that they become quantum mechanical in their behaviour,” he says. Transistor gate lengths are now about 22 nm, which is about 100 times the spacing of the individual silicon atoms. “There’s a concern about how small these devices can become before quantum effects take over, and this suggests they still have a few more generations,” Ferry adds.
Addressing single atoms
Simmons’ group, however, is not interested in conventional electronics and instead is working towards the development of quantum computers. The team hopes to use individual phosphorus atoms as quantum bit or qubits. “We’re developing single-atom devices,” explains Simmons, “and in that development we’ve realized that to be able to address a single atom, we need to be able to make the electrodes the same size – and that’s really what we’re using these wires for.”
On this point, Ferry is more sceptical not just about that approach but about quantum computing in general. “I’m considered to be one of the ‘antis’ in that world,” he says. Indeed, he even suggests that the persistence of classical phenomena on the atomic scale could make it difficult to use phosphorus atoms as qubits.
Nevertheless, Simmons remains optimistic. “Five years ago, there were lots of potential barriers to developing a phosphorus-based quantum computer and we’ve overcome those gradually, bit by bit. At the moment I guess the big challenge for quantum computing is to make a scalable system. Certainly these wires are very helpful towards that goal,” she says.
The research is published in Science.