
Heavy ion therapy is a promising cancer treatment technique, with beams of heavy ions delivering sharper Bragg peaks and less lateral scattering than a proton beam. But as tumour targeting gets more precise, it becomes increasingly critical to perform accurate verification of dose delivery. With this aim, researchers at National Institute of Radiological Sciences (NIRS) and Nagoya University in Japan have investigated the use of optical imaging for high-resolution range estimation in heavy ion beams.
The NIRS team used imaging of prompt luminescence and Cerenkov light to estimate the range of radioactive 11C and 15O ion beams created at the Heavy Ion Medical Accelerator in Chiba (HIMAC). They found that in-beam luminescence imaging can measure the Bragg peak, while offline Cerenkov imaging can determine the stopping position of the ion beams (Phys. Med. Biol. 10.1088/1361-6560/ab1ccf).
“The main benefits of optical imaging over other approaches being developed for beam range verification are its high resolution and low cost,” explains first author Han Gyu Kang. “The optical imaging is performed using a lens and a CCD camera, thus submillimetre spatial resolution can be achieved, which is hardly possible for PET or prompt gamma-based range verification. In addition, the optical imaging system costs less than $1000, while PET or prompt gamma systems require a number of scintillation crystals, photosensors and a complex signal processing unit.”

To assess their proposed optical imaging approach, Kang and colleagues irradiated a PMMA phantom with radioactive 11C and 15O ion beams (for 30 and 8 min, respectively) produced at HIMAC’s secondary beam line. They used a cooled CCD camera to measure the generated luminescence and Cerenkov light.
Since prompt luminescence light is only produced during irradiation, the researchers recorded luminescence images in-beam; Cerenkov images were obtained offline immediately after irradiation to exclude the luminescence signal. They recorded luminescence images for 4 and 2 min, and Cerenkov images for 30 and 2 min, for the 11C and 15O ion beams, respectively.
The longer Cerenkov imaging time required for the 11C ion beam is due to its relatively long half-life of 20 min, compared with 2 min for 15O. This imaging time could be reduced, say the authors, by replacing the current front-illuminated CCD camera with a back-illuminated CCD camera with higher quantum efficiency at 400 nm.
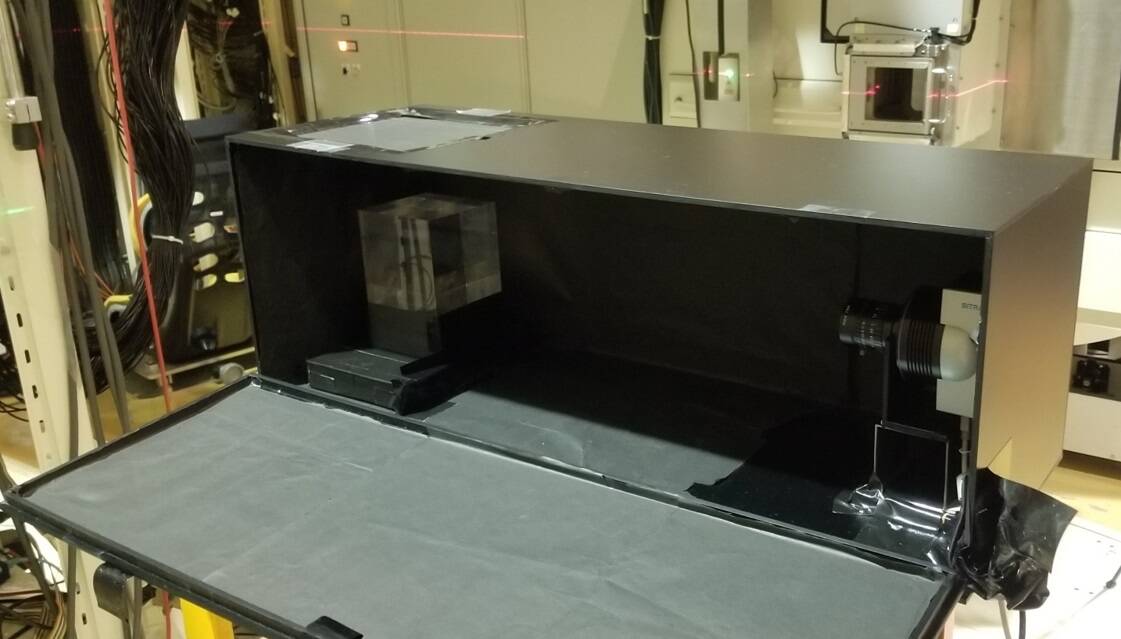
To validate the Cerenkov images, the team performed in-room PET, which maps positron emitters generated during irradiation. For both ion beams, the Cerenkov image had a similar shape to that of the PET image, indicating that Cerenkov imaging can visualize the stopping position of the ion beam as effectively as PET.
Comparisons of line profiles revealed that, for the 11C ion beam, the Bragg peak (at 44.0 mm) showed a good correlation with the peak of the luminescence profile (45.0 mm). Likewise, for the 15O ion beam, the Bragg peak (at 46.8 mm) corresponded well with the luminescence peak (47.2 mm).
The peaks of the Cerenkov profiles (50.6 and 49.7 mm, for 11C and 15O, respectively) matched the peaks of the PET profiles (50.5 and 49.5 mm). The authors note that the positional differences between the Bragg peak and the Cerenkov peak seen in both cases is due to the energy spread of the ion beam, which was larger for 11C ions.
For the 11C ion beam, the FWHMs of the line profiles were 16.0 and 18.1 mm, for the Cerenkov and PET signals, respectively. For the 15O ion beam, the respective values were 12.3 and 16.5 mm. These results demonstrate that Cerenkov imaging could provide better resolution than PET, the spatial resolution of which is limited by size of the scintillation crystal (typically 2 to 4 mm).
The authors conclude that optical imaging could be used to visualize the Bragg peak and stopping position of radioactive ion beams with sub-millimetre resolution. Due to the low penetration depth of optical photons, typically less than 10 mm, the technique cannot be used during patient treatment.
“Nevertheless, the optical imaging technique is a promising method for high-resolution and cost-effective Bragg peak measurement of radioactive ion beams,” says Kang. “Our next step is to investigate the feasibility of optical imaging for daily quality control of radioactive ion beams at NIRS.”