A new generation of extremely large telescopes requires fresh approaches to correct for the blurring effects caused by the Earth’s atmosphere, as Richard de Grijs, one of an international team of astronomers developing the ambitious Thirty Meter Telescope, explains
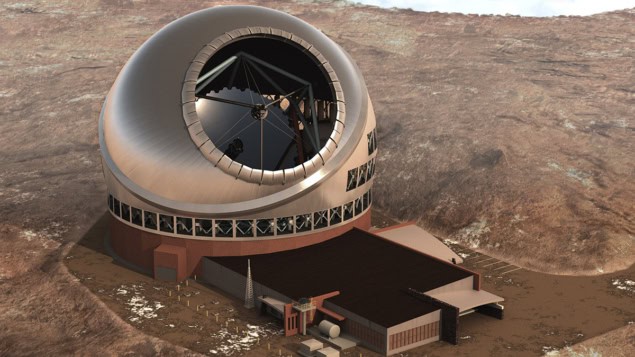
Astronomers around the world are attempting to outsmart the random nature of the Earth’s atmosphere, the part of our planet’s ecosystem that allows all of us to survive and thrive. A cool evening breeze may bring welcome relief after a long day in the suffocating summer heat, but that same gust of wind can wreak havoc on the feeble light signals that travel from distant stars and galaxies to reach telescopes on Earth.
The problem stems from turbulent eddies in the Earth’s atmosphere, which in turn are caused by changes in air density as the temperature fluctuates. Such atmospheric turbulence generates local variations in the refractive index of the air, which means that light from a cosmic object is refracted many times as it travels through the Earth’s atmosphere towards a telescope.
Unfortunately, telescopes that are designed to magnify starlight also amplify these atmospheric irregularities. If you were to take multiple photos of a star in quick succession, turbulence would cause a distorted image of the star to move around its position in the night sky. The buffeting effect of the wind also causes the telescope itself to vibrate – which is only made worse by imperfect mechanical couplings to the drive motors, the enclosure and other sources of vibration. As a result, long-exposure photos show a blurred stellar image rather than a sharp point source, with the size of the blurred image known by astronomers as “atmospheric seeing”.
Telescope designers have introduced optical techniques to correct for the effects of atmospheric turbulence, and these techniques have become ever more important and sophisticated as the light-collecting mirrors have become larger. The Thirty Meter Telescope (TMT) – now being developed in a collaboration between the US, Canada, Japan, China and India – is pushing this technology to new limits, with the ultimate aim of building a ground-based observatory with a spatial resolution more than an order of magnitude greater than that of the Hubble Space Telescope.
The first telescopes to correct for atmospheric effects exploited so-called active optical systems, which provide real-time control of the secondary mirror’s position relative to the main mirror. These active systems incorporate computer-controlled actuators positioned under the main mirror to constantly adjust its shape, helping to control the defects and deformations in the telescope structure, mirror and enclosure that are caused by temperature changes, wind buffeting and mechanical effects.
Such active optical systems greatly improve the quality of the resulting images, but telescope mirrors can only be adjusted at low frequencies, typically around 1 Hz. In contrast, atmospheric turbulence fluctuates on millisecond timescales.
New routes to sharper images
More responsive error correction can be achieved with adaptive optics (AO) systems, which use a reference star in the telescope’s field of view or an artificial laser “guide star” to continually sample the conditions that the light passes through. An optical device called a wavefront sensor analyses the incoming starlight, and prompts a computer to send corrective commands to small, deformable mirrors close to the telescope’s focus. Most telescopes with mirror diameters in the 8–10 m range are equipped with Shack–Hartmann wavefront sensors, in which the focal planes are covered with arrays of small lenslets. In this case, the wavefront distortion is measured for each lenslet, which in turn is fed back into the deformable mirror loop.
Most AO systems in operation today exploit a single guide star, but they can only correct the distortions over small patches of the sky. As an example, the bright
spot that can be generated by exciting sodium atoms at a height of around 90 km cannot sample atmospheric turbulence at greater heights. More importantly, the cone-shaped volume defined by the telescope mirror and the laser spot does not allow the AO system to sense the outer portions of the laser’s wavefront, which can cause differential stretching effects when using the laser guide star to correct the target’s wavefront.
The obvious remedy is to exploit multiple laser guide stars, which together can sample the atmosphere above the telescope more homogeneously. As a result, all current designs for the next generation of large telescopes include versions of such “multi-conjugate” AO systems.
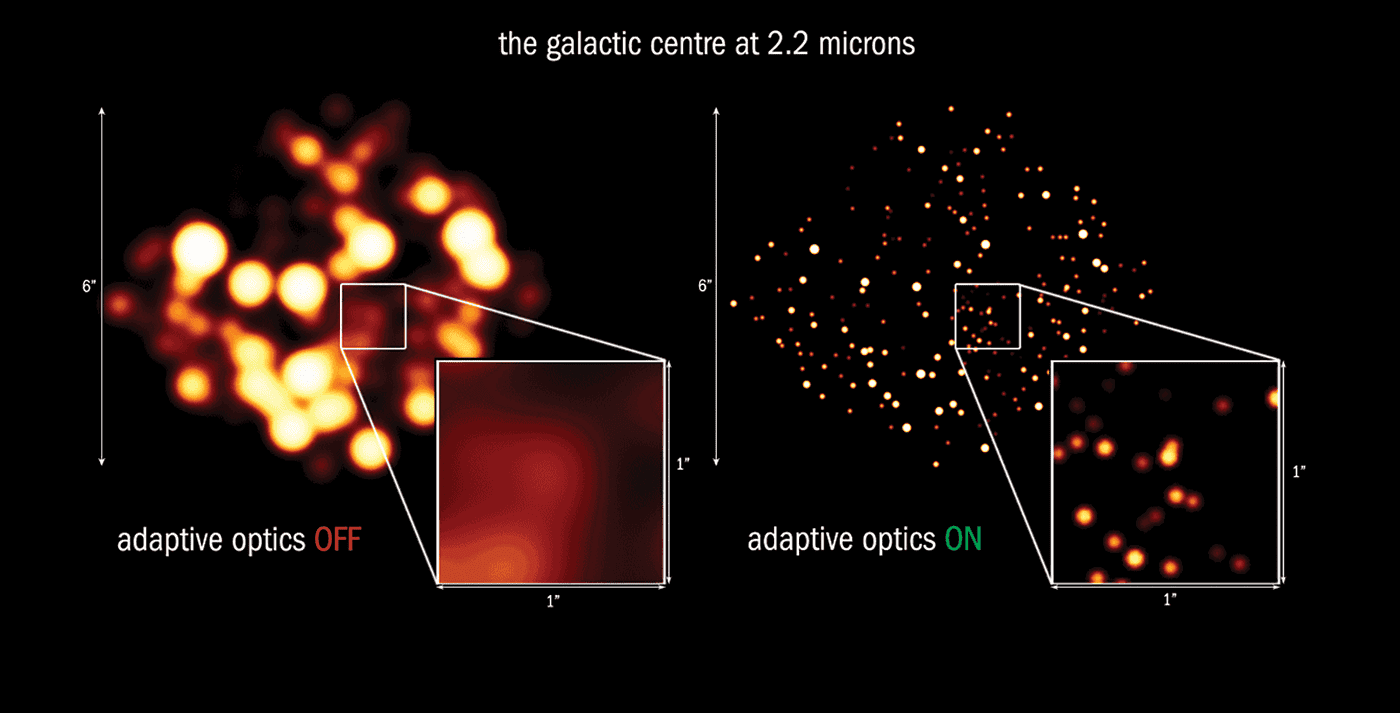
Meanwhile, the TMT is taking the AO concept one step further. Just like any other telescope, the TMT can be used as a huge “light bucket” to observe the night sky without any atmospheric correction. But it is also on track to become the first telescope designed with AO as an integral system element, part of a low-risk design strategy that will enable advances in the technology to be incorporated into the telescope as they are developed.
This integrated AO approach is crucial for the TMT to achieve its design goal, which is to routinely provide a diffraction-limited spatial resolution that will be 12.5 times sharper than that of the Hubble Space Telescope. At the diffraction limit the gains in sensitivity scale with the fourth power of the main mirror’s diameter, which means that the TMT will be two orders of magnitude more sensitive than the current generation of large telescopes.
Inaugural system
When the TMT produces its first images – most likely soon after 2027 – it will be equipped with a multi-conjugate AO set-up incorporating six laser guide stars and two deformable mirrors. This inaugural system, called the Narrow Field Infrared Adaptive Optics System (NFIRAOS), will provide diffraction-limited images over the 0.8–2.5 μm wavelength range, where AO offers optimal results.
The NFIRAOS is expected to reduce wavefront errors to well below 190 nm over a 10–30 arcsecond field of view, in so-called “median seeing”. Given the usual atmospheric conditions at the preferred construction site, Mauna Kea, the highest summit of the Hawaiian islands, this median seeing requirement implies excellent, highly competitive performance. Astronomers have been considering alternative sites as a result of sustained opposition from native Hawaiians, and have just confirmed La Palma in the Canary Islands as their second choice – which promises world-class performance, although not quite as good as on Mauna Kea.
In practice, the NFIRAOS is expected to improve the typical size of a point source from around 0.5 arcseconds in “seeing-limited” mode to about 0.01 arcseconds. A defined upgrade path is set to further reduce the wavefront errors to approximately 133 nm within five years, significantly better than the performance of any current AO system in operation. The TMT will then be blessed with an AO system that can deliver competitive Strehl ratios – a quantitative measure of how much of the light collected by the detector is delivered into the diffraction-limited point source – of 40–75% at wavelengths from 1.2 to 2.2 μm.
NFIRAOS will mostly be used to obtain near-infrared spectra – either single observations using a “long slit” or multiple targets simultaneously in “integral field unit” mode. Additional AO systems are also being developed for deployment within the first decade of TMT operations: one optimized for spectroscopic observations at mid-infrared wavelengths (small-field, diffraction-limited mid-infrared AO: MIRAO), and another for near-infrared correction of multiple small sky areas over a 5 arcminute field of view (multiple-object AO: MOAO).
Such diffraction-limited operation of 30 m class telescopes will enable a step change in our knowledge of the universe. But advances in technology must proceed in tandem with our scientific understanding for the TMT and its class of next-generation large telescopes to make ground-breaking discoveries well into the future.