Although they were discovered almost 20 years ago, photonic crystals are still proving to be a remarkably fertile area of physics research. In the past week researchers from the US and the Netherlands have made the first three-dimensional photonic quasicrystals, while another US team has used a photonic band-gap structure to accelerate electrons.
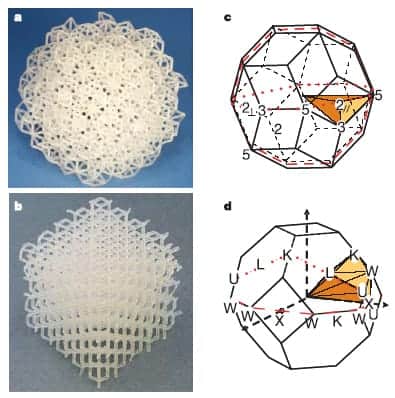
Photonic crystals are materials in which a periodic variation of the dielectric constant results in a photonic band-gap. This means that photons with wavelengths or energies in this band-gap cannot travel through the crystal. Quasicrystals, on the other hand, are materials that do not have a periodic lattice structure but still display subtle long-range order not possible in regular crystals. Researchers have already made one- and two-dimensional quasicrystals that have photonic band-gaps. Now Paul Steinhardt, Weining Man, Paul Chaikin and Mischa Megens of Princeton University, New York University and Philips Research Laboratories in Eindhoven have performed this feat in three-dimensional quasicrystals for the first time (Nature 436 993).
Deciding that it was too difficult to explore the problem theoretically or computationally, Steinhardt and co-workers decided to make a 3D icosahedral quasicrystal and see if it demonstrated a band-gap. They joined several thousand one-centimetre long plastic rods into a diamond crystal array – a structure known to have a photonic band gap – and shone microwaves at it. They found that light is trapped by the structure in certain planes, and that these planes enclose an almost spherical, 30-sided polyhedron called a triacontahedran (figure 1).
According to Steinhardt, quasicrystals are ideal candidates for making photonic band-gap structures that could be used in a variety of applications in computation and communication. The team is now exploring three different areas of research: the use of optical tweezers to make materials that have band-gaps at optical rather than microwave wavelengths; the use of rods spheres and surfaces – as opposed to just rods – to make the materials; and exploring different types of photonic, electronic and even acoustic applications.
Meanwhile, Evgenya Smirnova and colleagues at the Massachusetts Institute of Technology (MIT) have used photonic crystals to accelerate particles for the first time. This result could help researchers make a table-top particle accelerator that is capable of accelerating particles into the TeV range (Phys. Rev. Lett. 95 074801).
Smirnova and colleagues made a two-dimensional photonic crystal with a periodic lattice of metal rods and then converted it into a waveguide by removing a rod from the centre of the lattice (figure 2). When the waveguide was placed in a test beam at the MIT accelerator laboratory and powered with 2 megawatts of microwaves it increased the energy of a 16.5 MeV electron beam by 1.4 MeV. The electrons were accelerated as a result of interactions with high-frequency microwaves inside the waveguide.
The main advantage of the new approach is that it suppresses unwanted “wake fields” that are created by the electron beam. Wake fields are a problem for conventional accelerators because they lead to beam loss.
“Our photonic band gap waveguide is designed so that is only supports the particle accelerating modes and not any wake fields, thus making highly efficient acceleration possible,” says Smirnova. The MIT team now plans to build a bigger stand-alone photonic crystal accelerator and power it with more microwaves to increase the acceleration.