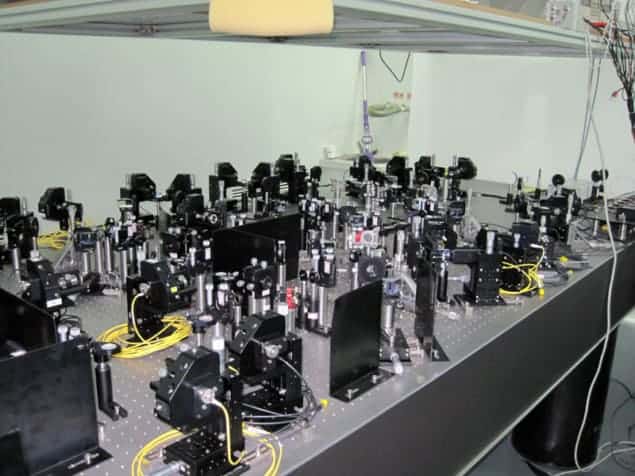
Physicists in China have broken their own record for the number of photons entangled in a “Schrödinger’s cat state”. They have managed to entangle eight photons in the state, beating the previous record of six, which they set in 2007. The Schrödinger’s cat state plays an important role in several quantum-computing and metrology protocols. However, it is very easily destroyed when photons interact with their surroundings, prompting the researchers to describe its creation in eight photons as “state of the art” in quantum control.
In Erwin Schrödinger’s famous thought experiment of 1935, all of the molecules in a cat are in a superposition of two extreme states – living and dead – and an observer cannot tell which until a measurement puts the cat into one of the two states. Today physicists use the term “Schrödinger’s cat state” (or Greenberger–Horne–Zeilinger state) to describe any multi-particle quantum system that is in a superposition of extreme states.
For example, a pair of entangled photons can be created in the lab such that they are in a superposition of both photons having horizontal polarization and both having vertical polarization. Entanglement is a quantum effect, which means that particles such as photons can have a much closer relationship than is allowed by classical physics. By measuring the polarization of one of the pair, we immediately know the state of the other, no matter how far apart they are.
The Schrödinger’s cat state of eight entangled photons was created by Jian-Wei Pan and colleagues at the University of Science and Technology of China in Hefei. The team began by firing laser light at a nonlinear crystal, which converts single high-energy photons into pairs of entangled lower-energy photons with perpendicular polarizations. The polarization of one of the photons was then rotated by 90°, which puts each pair into a two-photon Schrödinger’s cat state.
Pairing up photons
Pan and colleagues then took one photon from each pair and combined the quartet in an optical network consisting of three polarizing beam splitters. One photon leaves each of the network’s four outputs only if all four photons have the same polarization. As there is no way of knowing what this common polarization is, the photons are therefore entangled in a Schrödinger’s cat state. But as each of the four photons is already entangled with one other photon, all eight photons are therefore entangled in a Schrödinger’s cat state.
This entanglement was established by measuring the polarizations of the eight photons as they emerged from the experiment. This reveals the “fidelity” of the eight-photon Schrödinger’s cat state, which effectively says how close the different states are to the ideal Schrödinger’s cat. The team measured a fidelity value of 0.708 – much larger than the threshold value of 0.5, above which a state is considered to be entangled.
According to Xiao-Qi Zhou of the University of Bristol, UK, Pan and team were able to entangle eight qubits because they managed to separate the photons into “ordinary light” and “extraordinary light”. Both types are produced by parametric down conversion and ensuring that four extraordinary photons are sent for further entanglement boosts the efficiency of the process.
Hyper-entanglement could be next
Pan told physicsworld.com that there are several ways that the team can take this work forward. One is to use “hyper-entanglement” to create a 16-qubit Schrödinger’s cat state for their eight photons. Hyper-entanglement makes use of more than one degree of freedom of the photon – momentum and polarization, for example – which multiplies the number of states that can be entangled. In 2008 the team used hyper-entanglement to create a 10-state Schrödinger’s cat state using five photons.
Zhou points out that the technique of separating ordinary and extraordinary light could also be used to entangle six photons at a higher efficiency than previously possible. This, he thinks, could be used to create a wide range of different entangled states that could be used in quantum computing.
The Schrödinger’s cat state could be particularly useful for quantum error correction, which protects a quantum computation from the destructive effects of noise. For example, one bit of quantum information (a qubit) could be encoded into all eight photons of a Schrödinger’s cat state. If the polarization of one of the eight photons is inadvertently flipped, for example, this can be corrected by determining the value of the other seven photons.