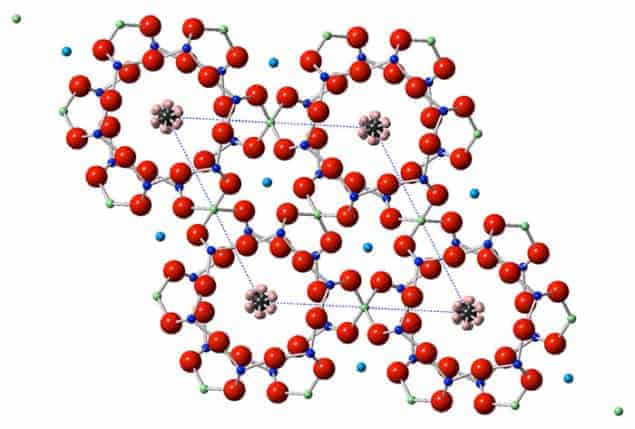
Water molecules trapped in tiny channels exist in a blurry quantum superposition of six different configurations that bears little resemblance to the structure of a free molecule. That is the finding of physicists in the US and UK, who have used neutron scattering to map the locations of hydrogen atoms in water molecules trapped in the mineral beryl – revealing that the atoms tunnel between the six configurations. The researchers have also found evidence that, unlike normal water, a trapped molecule has a zero electric-dipole moment. The research could shed light on how water behaves when confined to tiny spaces, such as in the membranes of living cells.
Tunnelling is a purely quantum-mechanical phenomenon whereby a particle can pass through an energy barrier without having enough energy to climb over the barrier. Researchers have already seen hydrogen atoms tunnel from one part of a molecule to another. In methyl and ammonia groups, for example, the tunnelling appears as a rotation of hydrogen atoms about a molecular axis. Now, Alexander Kolesnikov of the Oak Ridge National Laboratory and colleagues in the US and UK have spotted hydrogen atoms tunnelling in single water molecules that are trapped in tiny channels within the crystalline mineral beryl.
Tiny channels
The channels are about 0.5 nm wide, which means that they can only contain one water molecule at a time. Previous terahertz-spectroscopy studies suggested that rotational tunnelling of hydrogen was occurring in the beryl-trapped water, and now Kolesnikov and colleagues have measured the effect in two separate neutron experiments – one done at the Spallation Neutron Source (SNS) at Oak Ridge and the other at the ISIS neutron source at the Rutherford Appleton Laboratory in the UK.
At the SNS, the team fired a beam of low-energy “cold” neutrons at beryl-trapped water molecules. As they scatter, the neutrons can gain or lose energy by coupling to transitions between rotational states of the hydrogen atoms, leading to a neutron-energy spectrum with seven distinct peaks. The experiments were carried out at temperatures between 5–50 K, with the peaks shrinking as the sample warms. This is what would be expected if the peaks are associated with tunnelling, because as the temperature increases, the increased thermal motion of the atoms would wash out any quantum-tunnelling effects.
Six-fold symmetry
A beryl channel resembles a hexagonal tube, and the team reckons that a V-shaped water molecule (two hydrogen atoms attached to an oxygen vertex) will sit with its oxygen atom in the centre of the tube and its two hydrogen atoms pointing above and below the hexagonal plane (see image). There are six different ways that the V can be oriented, each separated by a rotation of 60°. While there is an energy barrier between each of these configurations, hydrogen atoms can tunnel from one orientation to the next, resulting in a spectrum of rotational states. Calculations done by the team show that seven peaks observed by neutron scattering correspond to transitions between these rotational tunnelling states.
The researchers were also interested in how localized the hydrogen atoms are, which they measured using deep inelastic neutron scattering (DINS) at the ISIS neutron source in the UK. By firing a beam of higher-energy neutrons at beryl-trapped water molecules and measuring the momentum transfer between the neutrons and individual hydrogen atoms, the team determined the momentum distribution and kinetic energy of the atoms. Kolesnikov and colleagues found that the kinetic energy of the atoms is about 70% of that measured in unconfined water. This, they conclude, means that the positions of the atoms are much more smeared out in the Beryl-trapped water than in normal water.
Writing in Physical Review Letters, the team concludes that the trapped molecules are “a new state of the water molecule” – one that is a blurry ring with six-fold rotational symmetry. Such a molecule, the team believes, would not have an electric-dipole moment. An unconfined water molecule has an electric dipole moment, which is responsible for water’s familiar properties such as its relatively high boiling point and its ability to dissolve many different substances. The new research could therefore provide important clues about how water behaves differently when it is confined to tiny spaces such as the membranes of living cells.