Some scientists claim they can control genetically engineered neurons using magnetic fields. Have they and the high-profile journals that published their research failed to understand basic physics? Edwin Cartlidge investigates
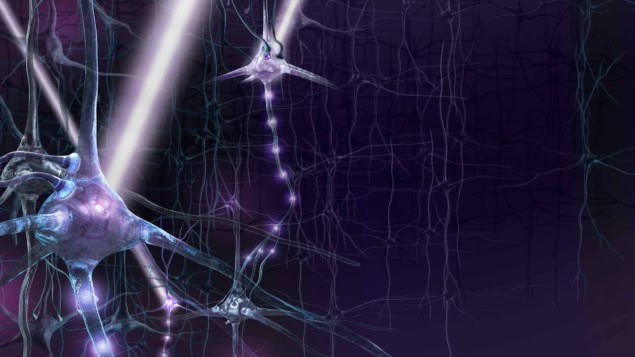
“Badass”. That was the word Harvard University neuroscientist Steve Ramirez used in a Tweet to describe research published online by fellow neuroscientist Ali Güler and colleagues in the journal Nature Neuroscience last March. Güler’s group, based at the University of Virginia in the US, reported having altered the behaviour of mice and other animals by using a magnetic field to remotely activate certain neurons in their brains. For Ramirez, the research was an exciting step forward in the emerging field of “magnetogenetics”, which aims to use genetic engineering to render specific regions of the brain sensitive to magnetism – in this case by joining proteins containing iron with others that control the flow of electric current through nerve-cell membranes.
By allowing neurons deep in the brain to be switched on and off quickly and accurately as well as non-invasively, Ramirez says that magnetogenetics could potentially be a boon for our basic understanding of behaviour and might also lead to new ways of treating anxiety and other psychological disorders. Indeed, biologist Kenneth Lohmann of the University of North Carolina in the US says that if the findings of Güler and co-workers are confirmed then magnetogenetics would constitute a “revolutionary new tool in neuroscience”.
The word “if” here is important. In a paper posted on the arXiv preprint server in April last year and then published in a slightly revised form in the journal eLife last August, physicist-turned-neuroscientist Markus Meister of the California Institute of Technology laid out a series of what he describes as “back-of-the-envelope” calculations to check the physical basis for the claims made in the research. He did likewise for an earlier magnetogenetics paper published by another group in the US as well as for research by a group of scientists in China positing a solution to the decades-old problem of how animals use the Earth’s magnetic field to navigate – papers that were also published in Nature journals.
In all three cases, Meister finds that the relevant magnetic interactions are between five and 10 orders of magnitude too weak to account for the claimed effects. As such, he concludes, the “claims are in conflict with basic laws of physics”. He adds: “If the reported phenomena do in fact occur, they must have causes entirely different from the ones proposed by the authors.”

The three groups under attack have defended themselves by arguing that experiment must trump theory, particularly in the messy world of biology. But Meister insists that it is vital to offer a viable physical explanation of the purported effects because, he argues, claims that violate the laws of physics often turn out to be wrong – as was the case, he says, with the idea of “water memory” put forward by French immunologist Jacques Benveniste in the 1980s to explain homeopathy.
For Meister, the issues at stake regard the sociology of science as much as they do the science itself, particularly what he sees as high-profile journals’ unhealthy appetite for eye-catching, as opposed to robust, research. He criticizes the authors for not having carried out simple calculations to check their results, but above all takes aim at the journal editors and reviewers, who he argues need a far more rigorous approach to vetting submitted manuscripts. For the research in question, he maintains, their level of quality control “borders on malpractice”.
The attraction of magnetism
Doctors currently use a number of electrical and magnetic techniques for stimulating the brain in order to treat psychological, neurological and other conditions. However, each technique has its down-sides, such as the need to place electrodes inside people’s skulls and the inability to target specific groups of neurons. But in the meantime scientists have been using genetic engineering to try to improve targeting. One option is to make neurons sensitive to certain drugs, but this method tends to be slow-acting and imprecise. A quicker, more accurate alternative, known as optogenetics, involves controlling neurons using light, but this requires optical fibres to be inserted into the brain.
The virtue of a magnetic technique lies in magnetic fields’ very weak interaction with biological tissue, which means they can penetrate deep inside the brain unguided by wires, fibres or any other conduit. But this weak interaction is also a curse as it makes intercepting fields where required very hard to do. Nevertheless, Güler’s group reckoned it had found the answer by fusing two proteins together. One of these, known as ferritin, is paramagnetic, which means that it becomes magnetized when placed in an external magnetic field. The other protein, called TRPV4, acts as an “ion channel” across cell membranes – a “gateway” for ions, which can be opened or closed by mechanical forces. In the presence of a static magnetic field, the researchers reasoned, the magnetized ferritin would “tug open” TRPV4’s central pore and allow an electric current to flow out of nerve cells.
In their paper (2016 Nature Neurosci. 19 756), Güler and colleagues list numerous lines of experimental evidence to back up their claims. For example, they report delivering a DNA sequence, containing the gene encoding its TRPV4–ferritin complex, into zebrafish larvae (targeting trunk and tail neurons), and finding that they could make the larvae coil by placing them in a magnetic aquarium. The researchers also showed they could entice mice into a magnetic chamber by giving them a virus with the same gene, but which was instead targeted at a region of the animals’ brain containing dopamine-releasing neurons.
This research followed an earlier published article (2015 Nature Med. 21 92) – one of the other two papers Meister has scrutinized – in which geneticist Jeffrey Friedman of Rockefeller University in New York and colleagues described how they instead fused ferritin to TRPV1, an ion channel that responds to changes in temperature. The idea here was to use a high-frequency magnetic field to continually flip the spins of the constituent iron atoms so that they dissipate heat when dropping back to their equilibrium position. The resulting rise in temperature would then open the ion channel and allow a current to flow.
Friedman’s group reports the results of several experiments, including one in which it genetically engineered stem cells and viruses in order to deliver genes encoding the TRPV1–ferritin protein complex, together with genes encoding insulin, into diabetic mice. Subsequently exposing those mice to either radio waves or a magnetic field reduced their blood sugar levels, the researchers found.
Lacking moments
These papers are not the first in which scientists have reported being able to switch neurons on and off by exposing iron-based nanoparticles in the brain to magnetic fields. Among others, both Friedman’s group (2012 Science 336 604) and one at the Massachusetts Institute of Technology led by materials scientist Polina Anikeeva (2015 Science 347 1477) reported activating TRPV1 magnetically. However, they did so using synthetic nanoparticles made from the iron oxide magnetite.
Because these particles are made outside the brain and then fed into it – in the case of Anikeeva’s group, simply using a syringe – they cannot be easily targeted at specific types of neuron. The research scrutinized by Meister is designed to overcome this problem by using genetic engineering to enable the creation of magnetic particles within brain cells. However, those particles – ferritin – are magnetic tiddlers. Each magnetite particle measures at least 20 nm across and contains several hundred thousand closely packed iron atoms, which interact strongly with one another and give the magnetite an inherent magnetic moment. Ferritin, in contrast, is a spherical shell surrounding a roughly 5 nm-diameter core containing just a few thousand, loosely packed iron atoms that lack the mutual interaction needed to generate a magnetic moment in the absence of an external field.
In his analysis, Meister makes a series of calculations showing ferritin to be completely unsuited to magnetogenetics. He points out that ferritin’s “induced” moment is proportional to the strength of the external field that is applied, and as such is many orders of magnitude smaller than that of magnetite.
1 Forces at play
Physicist-turned-neuroscientist Markus Meister critically examined four possible scenarios in which a magnetic field could be used to induce magnetic moments in ferritin particles (green) in order to open the ion channel TRPV4 (pink). He calculated the forces created in each case, concluding that they would all be too weak to open TRPV4. The magnetic field B induces a moment m in the ferritin core, leading to a force F or a torque N on the ferritin particle, with the resulting forces tugging on the channel.
In Meister’s paper (2016 eLife 5 e17210), he first works through four scenarios (figure 1) in which a magnetic field could impart a mechanical force on the ferritin, concluding, contrary to the claim by Güler’s group, that any such force would be far too puny to open TRPV4. He shows that the force created by a field gradient pulling on the ferritin (figure 1a) would be nine orders of magnitude smaller than that needed to open the (well-studied) ion channels in auditory hair cells, while the interaction between neighbouring ferritin particles (figure 1b) would fall short by eight orders of magnitude. To be on the safe side, he also calculates an absolute thermal limit in the second scenario. (This is a measure of how much the system is jiggling thanks to its thermal energy. For an effect to be significant – a signal, rather than noise among the jiggling – it has to be of similar or larger magnitude.) But Meister reaches a similar result: the energy imparted by the field would be about 100 million times smaller than the ion channel’s thermal energy.
Multiple ferritin particles pulling on a cell’s membrane would generate a stress at least a million times smaller than that needed
Meister finds that even the best of the four scenarios, in which ferritin feels a torque by virtue of being more easily magnetized along a certain axis (figure 1c), would see magnetic energy four orders of magnitude smaller than thermal energy. Last, he calculates that multiple ferritin particles pulling on a cell’s membrane (figure 1d) would generate a stress at least a million times smaller than that needed to deform the membrane so as to create ion channels across it.
In then analysing the results from Friedman and colleagues, Meister explains that magnetic heating of nanoparticles becomes very inefficient for particles below about 10 nm in diameter. Indeed, he says that previous research has shown ferritin’s “specific loss power” – the heating power generated per unit mass of material – to be “too low to be measurable”. To “keep the argument alive”, as he puts it, he instead considers the heat given off by an artificial form of ferritin filled with cobalt-doped manganite instead of straightforward iron.
He calculates that the temperature rise on the material’s surface in Friedman’s experiments would be a mere 10–10 K – more than 10 orders of magnitude less than the roughly 5 K needed to activate a TRPV1 ion channel. As a further check, he works out the overall effect on a nerve cell due to the combined heating of 10,000 ferritin particles crammed onto the cell’s surface. Here too the temperature rise would be minuscule – about 10–9 K.
For Meister, these disparities rule out any possibility that the effects claimed by the two groups are caused by magnetic interactions. “The discrepancy in these cases is like claiming to have built a perpetual motion machine,” he says. “Even the patent office no longer accepts such claims because they agree that we understand the physics well enough to dismiss them.”
Off course
Meister only penned his critique of the magnetogenetics research after having first read the paper on animal navigation, which was published by Xie Can of Peking University and colleagues in Nature Materials online in 2015 (15 217). As he puts it, the two subjects are “closely linked, because uncovering nature’s method for magnetosensation can point the way to effectively engineering magnetogenetics”.
He says that he immediately knew the paper by Xie and co-workers “was wrong”, having worked on the navigation of bacteria as a PhD student under bio-physicist Howard Berg. He explains that only in the case of what are known as magnetotactic bacteria is it well understood how an organism can sense and make use of the Earth’s weak magnetic field. These creatures act like tiny compass needles by synthesizing and lining up crystals of magnetite, which allows them to follow magnetic field lines down to their preferred habitat – the muck at the bottom of ponds.
The proposal by Xie’s group also involves a compass-needle like structure, but in this case the structure consists of a single molecule: a rod formed from an iron–sulphur protein surrounded by light-sensitive proteins. The researchers claim that the molecule has an intrinsic magnetic moment, having observed its orientation parallel to the Earth’s magnetic field using electron microscopy. They also report that the genes encoding the magnetic core are found in many animal species.
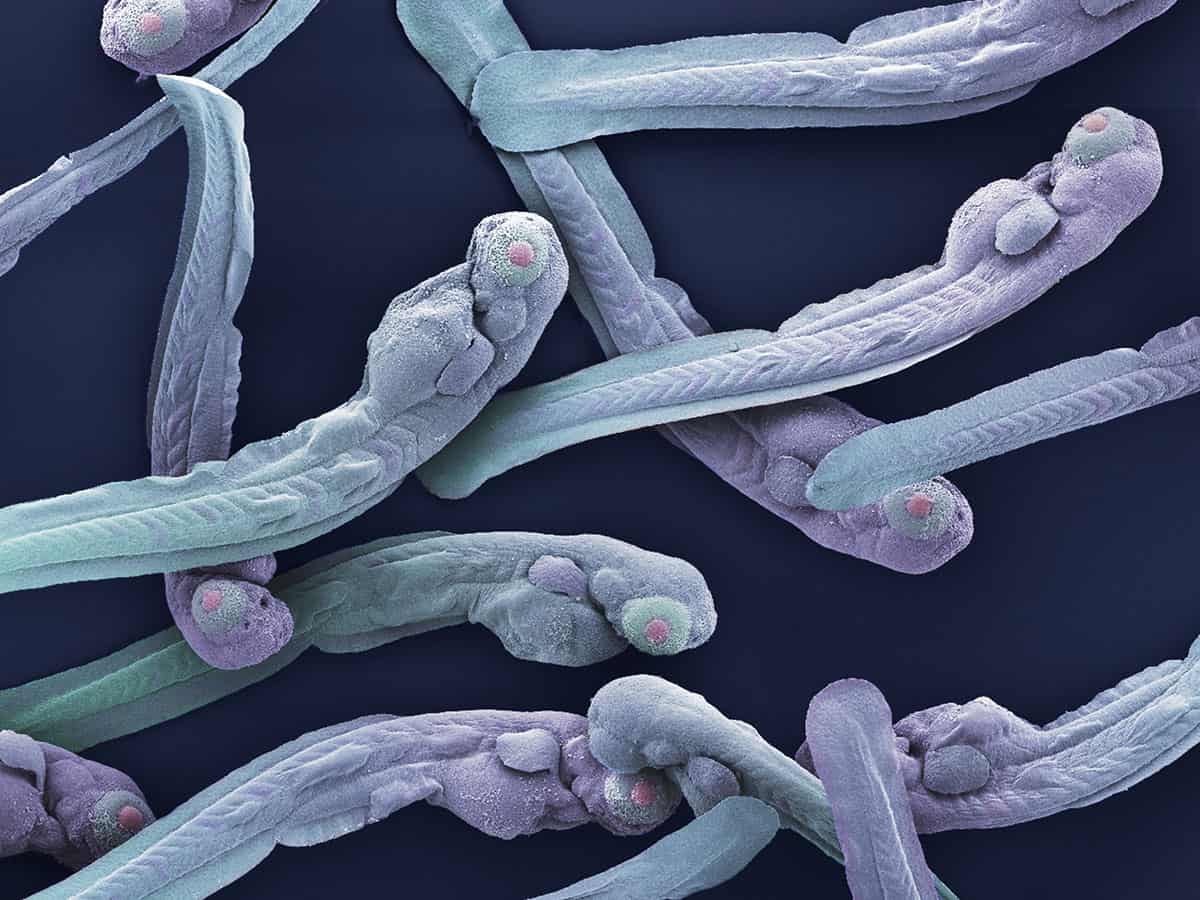
However, as Meister points out, the magnetism of the iron–sulphur protein would be puny even by the standards of ferritin – the molecule contains a mere 40 iron atoms spread out over 24 nm. At any temperature above a few degrees kelvin, he argues, the atoms’ thermal energy would randomize their spin directions, so preventing the formation of a magnetic domain and with it a permanent magnetic moment. He goes on to work out that even if the 40 atoms did somehow manage to form a domain, there is still no way they could line up with the Earth’s magnetic field because their interaction with it would be 100 000 times weaker than their thermal motion. “Clearly the reported observations must arise from some entirely different cause, probably unrelated to magnetic fields,” he writes.
Searching for wiggle room
In responding to Meister’s criticisms, all three groups insist on the primacy of experimental results. In a written statement sent to eLife, the two American groups in his cross hairs argue that “the intrinsic complexity of biologic processes” can limit the utility of theoretical calculations, and assert that “mathematical theory needs to accommodate the available data, not the other way around”. They express surprise that Meister would “stridently question” the validity of the two independent data sets without carrying out any experiments of his own.
As to exactly what they think is responsible for their observations, they won’t say. They give no hint in their written statement – simply saying that “the precise mechanism is undetermined” – and declined to answer more specific questions regarding this and other aspects of their work put to them by Physics World. The one thing that Friedman did add, via e-mail, was that he and the rest of his group “do not believe this [i.e. the mechanism in their experiment] to be a thermal effect”.
Xie, meanwhile, argues that it is “extremely challenging” to do simple calculations on the complex system he and his colleagues have studied. He maintains that the light-sensitive proteins can regulate the magnetic sensitivity of the iron–sulphur core, and that therefore the system’s overall magnetic moment cannot be calculated by considering the properties of the 40 iron atoms in isolation. “The data are what they are,” he says. “All we have to do is to interpret it carefully, or find an answer for it.”
Lohmann points out that physicists have sometimes been mistaken in the past when making elementary analyses of biological phenomena, most notably when dismissing the very idea that animals could detect the Earth’s magnetic field. In the 1960s they had argued that the terrestrial field interacts too weakly with biological tissue to be detectable, but Lohmann says that almost all scientists now accept the reality of animals’ magnetic sense. He notes that there are two different mechanisms that could at least in principle provide the sensitivity needed, one involving interactions with magnetite and the other relying on extremely complex chemical reactions featuring pairs of free radicals. “In that case simple back-of-the-envelope calculations yielded incorrect answers because the phenomenon involved mechanisms that people hadn’t thought of,” he says.
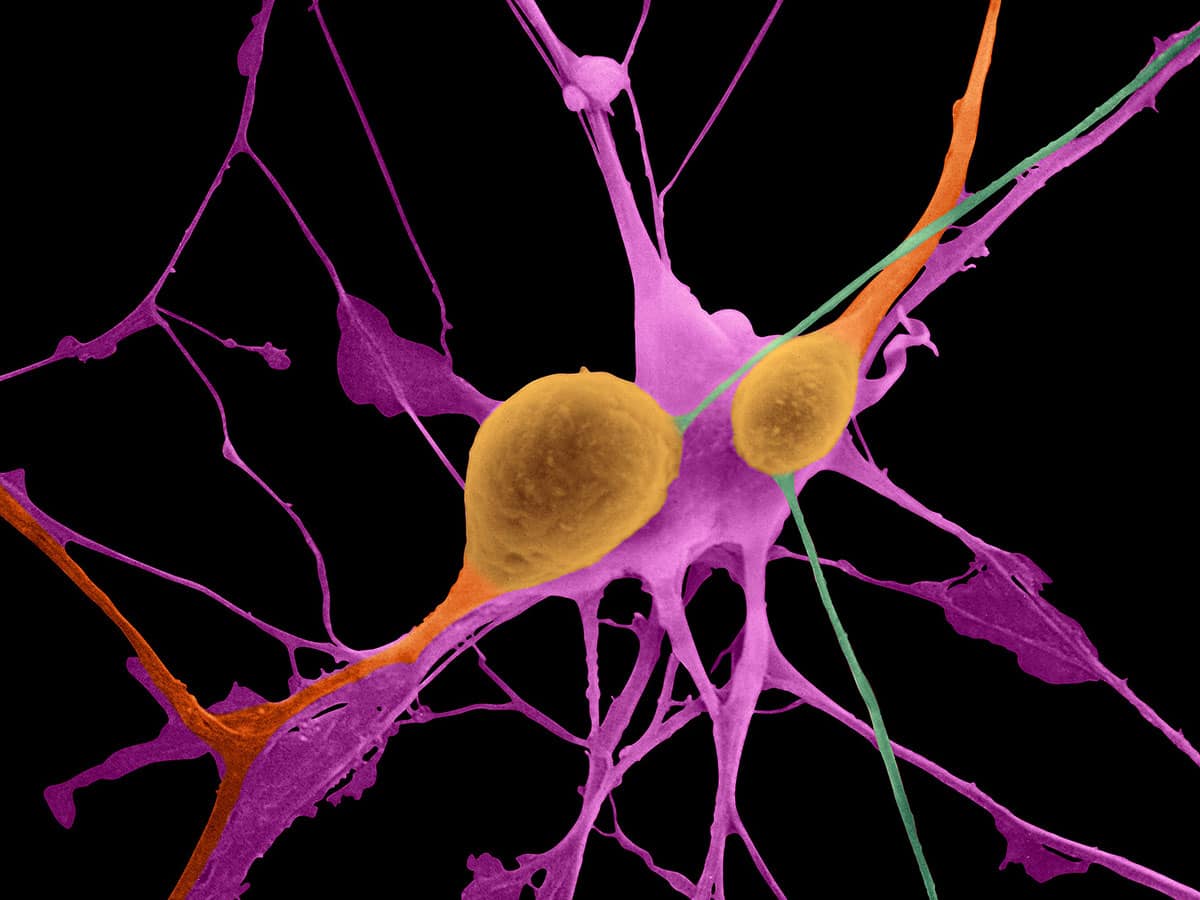
However, Lohmann maintains that the current dispute is a little different. He says that “time will tell” who is right, but argues that the magnetic interactions being claimed by the two American groups are “fairly straightforward” and adds that much is known about the forces needed to open ion channels – circumstances, he says, that “leave less room for unknown factors to complicate the analysis”. He also says that questions remain about the proposed biocompass put forward by Xie’s group, particularly whether such a structure actually exists in any animal and just how the protein complex would convert magnetic-field information into an electrical signal that could be interpreted by the brain.
Irreproducible, so far
Another person critical of the three papers is Anikeeva. She co-authored an article with fellow MIT researcher Alan Jasanoff for eLife endorsing Meister’s critique, and argues it was “premature” of the various groups to claim a magnetic origin for the effects they observed (2016 eLife 5 e19569). She also says that Friedman and colleagues are wrong to state that they stimulated neurons with radio waves, pointing out that the kind of solenoid they used to generate alternating magnetic fields creates negligible electric fields. “These devices don’t produce radiation,” she says.
(Friedman’s colleague Jonathan Dordick, a bio-chemical engineer at the Rensselaer Polytechnic Institute in upstate New York, replied in an e-mail that their device “creates an electromagnetic field, which by definition generates radiation”. He added “Whether or not one refers to this as radio waves is a semantic issue that does not alter our experimental results.”)
On the research discussion website PubPeer, meanwhile, people have taken aim at the experimental procedures and statistical analyses employed by the three groups, particularly those of Güler. One anonymous user argued that Güler and colleagues had failed to disentangle the effects on zebrafish larvae of magnetism and bright light, given that the latter, which is also known to induce coiling, appeared to be present only when the magnet was switched on. (Güler responded in an e-mail by saying that the difference in lighting was only apparent and was caused by reflections when the magnets were present.)
To encourage others to try and replicate their results, Güler distributed copies of DNA used by his group to a number of other scientists. But as Physics World went to press, there had been no reports of anyone else confirming the claims of his group or those of the other two. Indeed, Meister says that he knows of several other groups that have tried to reproduce one or other of the claimed results but that none has so far succeeded.
Meister praises Güler for stimulating further experimental work, but believes that the original research should never have been published in the first place. He acknowledges that biologists might not have time to “keep their physics skills sharp” but argues that they should at least thrash out their ideas with physicist colleagues. Had they done so and, as a result, decided not to go ahead with their experiments, he maintains “they would have saved them-selves and would-be imitators a lot of time”.
Above all, however, Meister blames the journals. He says there is good evidence that a “large fraction” of all papers published in high-profile journals are “wrong or irreproducible”, in part, he maintains, because their editors “thirst for ‘novelty’ ”. He also says that he has had little joy previously in persuading editors to publish corrections or commentaries on questionable research. “Journals have very little interest in drawing attention to critiques of great stories that they have published before,” he says. “These things get swept under the rug as much as possible.”
(Physics World sent e-mails to the chief editors of the three journals that published the disputed papers but got no response besides that of a spokesperson for their publisher, Springer Nature. The spokesperson said that for “confidentiality reasons” staff were not able to comment on the “editorial history or review process” of any paper published in a Nature journal.)
Future promise
Although Meister and the other critics lament what they see as the three groups’ misunderstanding of basic physics, they nevertheless underline the importance of continued research in the field. Anikeeva says she is “prepared to give the benefit of the doubt” regarding Friedman’s and Güler’s experimental results, arguing that other groups should carry out experiments of their own to try and understand just what the underlying physical mechanisms could be. Her group will do its bit, she says, by systematically exposing iron-containing proteins to different combinations of magnetic field, illumination and chemical conditions.

And while Meister retains that for the most part ferritin’s induced magnetic moment is too feeble to enable practical realizations of magnetogenetics, he does think that one of the mechanisms he scrutinized could be used in the future. He estimates that a magnetic field of about 5 Tesla – some 100 times larger than that used by Güler’s group – might be big enough to stimulate membrane currents by exploiting ferritin’s anisotropy, adding that stretching ferritin proteins into rod-like shapes would enhance the effect. More generally, he says, replacing ferritin with particles possessing a permanent magnetic moment, such as bacterial magnetite, “may offer a physically realistic route to magnetogenetics”.
It is this potential for making magnetogenetics a practical proposition that renders publication of the three controversial papers “unfortunate”, argues Meister. “Now that the prize for magnetogenetics has seemingly been taken, what motivates a young scientist to focus on solving the problem for real?” he asks. What can help, he maintains, is peer review of papers after they have been published, via websites such as PubPeer. That can “reopen the claimed intellectual space for future pioneers” he writes.
Meister accepts the possibility that the experimental data obtained by Güler, Friedman and their colleagues are in fact correct, and that, if so, the researchers may have stumbled across new physics. That would be “fantastic if true”, he says, “because it would fundamentally change our understanding of nanoscale matter” and, he adds, undoubtedly earn them a Nobel prize. But he reckons the odds on that are slim, particularly given the amount that scientists already know about very small-scale magnetism thanks to industry’s push for ever higher density disk drives. “I would appreciate being wrong but unfortunately I don’t think that is going to happen,” he says