What happens when you ask some of the world’s leading physicists seven questions about the past, present and future of physics?
“Oh no, not another survey.”
“What a good idea.”
“These answers are off the top of my head, but then so are your questions.”
These were just three of the comments that Physics World received when it decided to conduct a survey of physicists for this special millennium issue. We faxed and e-mailed a list of seven questions to over 250 physicists around the world. Some of the questions were direct: name the five physicists who have made the most important contributions to physics. Others were more general and open-ended: would you study physics if you were starting university this year? The answers were sometimes predictable, sometimes surprising, often stimulating and, on occasion, philosophical.
In asking who were the greatest physicists, we were deliberately vague about whether we were referring to the past 100 years, the past millennium, or simply all of time. If we had restricted ourselves to the 20th century, we would have overlooked Newton and Galileo, all the great physicists of the 19th century, and the discovery of X-rays, radioactivity and the electron in the late 1890s. And if we had restricted ourselves to the past millennium, we would have ignored Aristotle, Archimedes and others.
In the end we received some 130 replies. Most were enthusiastic, some were self-deprecating or doubtful, and a few were humorous. The biggest problem in physics is “getting tenure or quantum gravity” joked one astrophysicist. Some people were too busy to reply, others disagreed with the idea of ranking physicists or discoveries, and others just disagreed with us altogether: “Your questions are ridiculous,” replied one eminent surface scientist. Only two respondents pointed out that the millennium really comes to a close at the end of next year.
The choice of names was arbitrary in that we selected people who, in our opinion, either think deeply about physics, who look beyond the confines of their own research, or who always have something thought-provoking to say. At the same time we tried to strike a balance between theorists and experimentalists, between men and women, between different disciplines within physics, and among the different countries of the world. We chased and chased to achieve as fair a balance of opinions as possible but there are, inevitably, still some countries and areas of physics that are under-represented.
“I am not convinced at all by your choice of questions, they will probably lead to incoherent answers,” wrote one recent Nobel laureate, who declined to answer the questions. We are afraid that we disagree with him, and hope that you – when you have read the findings of the survey – will do so too.
The questions
Q1. What have been the three most important discoveries in physics?
Q2. Which five physicists have made the most important contributions to physics?
Q3. What is the biggest unsolved problem in your field?
Q4. What is the biggest unsolved problem in the rest of physics?
Q5. Would you study physics if you were starting university this year?
Q1. What have been the three most important discoveries in physics?
Words like “important” and “discovery” may be dangerous as far as historians and sociologists of science are concerned, but the vast majority of physicists in our survey had no qualms about answering our first question. Time and time again three key discoveries were singled out: quantum mechanics, Einstein’s special and general theories of relativity, and Newtonian mechanics and gravitation. “In each of these three cases, the discovery in question not only revolutionized the branch of physics that it nominally addressed, but also provided a framework so deep and universal that all subsequent theories in physics have been formulated within it,” said quantum-computation pioneer David Deutsch of Oxford University.
String theorist Michio Kaku of the City University of New York was even more clear-cut: “The sum total of our physical knowledge of the universe is contained in two theories: relativity and quantum theory. This is the crowning achievement of 2000 years of investigation into the universe, since the time of the Greeks.”
Newton’s laws of motion and gravity were selected because together they represented the first major attempt to create laws of physics that can be expressed in mathematical terms and tested by experiment. They also overturned the long-held belief that heavenly bodies obey different principles to those on Earth. “Newton set the pattern for all of us to follow,” said Bernard Schutz from the Max Planck Institute for Gravitational Physics in Potsdam, Germany.
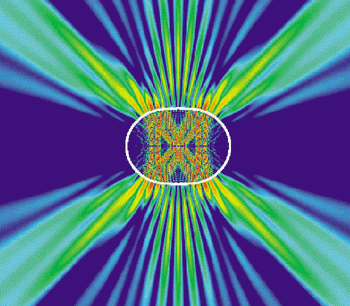
Einstein’s theories of relativity, on the other hand, showed that our intuitive understanding of physical quantities can be challenged at every level. “Part of the wonder of science is its ability to peel away layers of common intuition to reveal the true nature of our universe – to reveal features that are remarkable, stunning and sometimes rather distant from our day-to-day experiences,” enthused string theorist Brian Greene from Columbia University. “The special and general theories of relativity completely overturned previous conceptions of a universal, immutable space and time, and replaced them with a startling new framework in which space and time are fluid and malleable.” Einstein’s theories also have practical applications: for example satellite-based global positioning systems, which are widely used for navigation on the Earth, have to take general relativistic effects into account.
The other hugely popular choice, quantum mechanics, was dubbed “the most radically revisionist physical discovery of all time” by the physicist and Anglican priest John Polkinghorne. Or, as astrophysicist Piet Hut of the Institute for Advanced Study in Princeton explained: “Quantum mechanics completely overturned the classical notions of causality, objectivity and repeatability of experiments, introducing instead a form of spontaneity intrinsic to the natural world.” Many respondents also emphasized that quantum mechanics is not only elegant and powerful, but outstandingly useful as well. After all, quantum theory led to the development of semiconductors, transistors, lasers and – some might say – the entire microelectronics industry. It is also central to the design of new drugs and materials.
Maxwell’s unification of electricity and magnetism was another popular choice, because it was the inconsistency of electromagnetism with Newtonian physics that led to the development of special relativity. Electromagnetism also gave birth to the idea of fields, which have had “a huge impact, both from a practical and conceptual point of view” according to Daan Frenkel of the FOM Institute for Atomic and Molecular Physics in Amsterdam.
The realization that all matter is made of atoms was also mentioned many times. “I agree with Richard Feynman,” said Colin Humphreys, head of materials at Cambridge University, “when he said that if we could pass on just one sentence encapsulating the most important scientific knowledge we have, that sentence would be: ‘All things are made of atoms.’.”
Many respondents, however, chose individual moments of discovery that paved the way for new revolutions in physics. David Awschalom from the University of California at Santa Barbara, for example, selected Planck’s discovery of the quantum nature of light: “It was the first recognition of the fundamental inadequacy of classical physics. That was the hard part of quantum theory.”
Meanwhile, Lydia Iconomidou-Fayard of the Linear Accelerator Laboratory in Orsay, near Paris, chose the discovery of radioactivity: “It was the starting point for nuclear and high-energy research, and completely modified the view that people had of matter.”
Others chose the expansion of the universe, which led to the birth of modern cosmology, the invariance of the speed of light, which paved the way for relativity, and Thomson’s discovery of the electron – “the first fundamental particle and, unlike many fundamental particles discovered thereafter, incredibly useful” according to Humphreys. Rutherford’s discovery of the atomic nucleus was also selected several times, as was the discovery by Francis Crick, James Watson, Maurice Wilkens and others of the structure of DNA. The most recent discovery to receive a mention was last year’s evidence for neutrino mass, which was selected by astrophysicist Andrea Ghez of the University of California at Los Angeles.
Indeed, it is as one contemplates recent events that one realizes why historians and sociologists of science regard “discovery” as such a dangerous word. It suggests that the messy, complex process of science can be neatly packaged into individual breakthroughs, and implies that some sort of “ultimate truth” is out there, waiting to be unearthed. Even the theoretical physicist Paul Davies was not keen on the word, which he pointed out is often used by theorists to refer to the “invention” of a model. “Stephen Hawking is said to have ‘discovered’ that black holes are not black, but emit thermal radiation,” explained Davies. “Well, he didn’t. He discovered a mathematical model that predicts that. So are Newton’s laws of motion a discovery, or an invention, or what?” And if a phenomenon is predicted, rather than observed “out of the blue”, can that be called a discovery?
The word “important” can also be interpreted in many different ways. The discovery of quarks, for example, was important for theoretical physics, but it can hardly be said to have had much impact on everyday life. And to what extent is our judgement of importance affected by other factors, such as what we were taught in lectures as undergraduates? Some respondents therefore preferred to choose discoveries – or perhaps we should say “inventions” – that have had the most impact on society. Among those cited were the laser, the transistor, the telescope, the atom bomb and the scanning tunnelling microscope.
But the most unusual selection was made by Antonino Zichichi, a particle physicist at CERN, who proposed his own unique way of ranking the three most important discoveries. Zichichi selected three discoveries for which “the ratio of relevance divided by worldwide recognition is a maximum”. These were the discovery by Galileo that force is proportional to acceleration and not to speed, the discovery by Galvani that placing copper and zinc together produces electrical currents, and the discovery of “strange particles” by Clifford Butler and George Rochester at Manchester in 1947.
Q2. Which five physicists have made the most important contributions to physics?
“Some scientists are great because they are good all-rounders. Others make a major discovery by accident, but are not especially brilliant – just lucky. Others are brilliant, but never have a big discovery, although they can be immensely influential behind the scenes.” It was with these words of warning from Paul Davies that we added up the answers to this question in the Physics World office.
A total of 61 physicists received at least one vote, but it will come as no surprise to see Albert Einstein at the top of our list with 119 votes (see below). Einstein’s development of the special and general theories of relativity changed physics forever by revolutionizing the way in which we view space and time. Even one of his other “lesser” achievements, such as the explanation of the photoelectric effect, would have been enough to secure his reputation as one of the leading scientists of all time.
In second place with 96 votes is Isaac Newton – the man whose laws of mechanics and gravitation form the basis of vast swathes of classical physics, and who contributed much to the fields of optics, light and heat. Newton may have received fewer votes than Einstein because some respondents preferred to restrict their choices to scientists from the 20th century. Others felt that Galileo (6th in the list) deserved credit for paving the way for Newton’s discoveries.
In third place is the Scottish physicist James Clerk Maxwell, who expressed in his four famous equations two centuries of experimental discoveries in electricity and magnetism, and who successfully unified the two phenomena into one – electromagnetism. Although he mistakenly believed that electromagnetic radiation was carried through an invisible “ether”, Maxwell’s equations still remained valid even when Einstein’s theories disproved the notion of an ether. Maxwell also played a key role in the development of the kinetic theory of gases, as did his contemporary Ludwig Boltzmann (joint 11th), who laid the foundations of statistical physics, devised the notion of “entropy”, and did much to show that all matter is made from atoms.
The top 15 includes five physicists who worked on the development of quantum mechanics in the early part of the 20th century – Niels Bohr (4th), Werner Heisenberg (5th), Paul Dirac and Erwin Schrödinger (joint 8th) and Max Planck (11th). However, many respondents found it hard to select one individual from this group. Nobel prize winning particle theorist Steven Weinberg, who himself received two votes, went for Schrödinger. “He is really a stand-in for all the physicists who contributed to the discovery of quantum mechanics. I chose Schrödinger because it is his approach that turned out to be most useful.”
Although Dirac successfully developed relativistic quantum theory and predicted antimatter, it is Bohr who comes top of this sub-group – his 47 votes putting him 4th in the Physics World list. Bohr realized that the orbits of electrons in an atom are quantized, and although he stuck to his “semi-classical” view of the atom for many years, he inspired both Heisenberg and Schrödinger in the development of the matrix- and wave-mechanics versions of quantum theory. Bohr also contributed to the philosophical implications of physics, although his “Copenhagen interpretation” of quantum mechanics, which many physicists felt was the final word on the matter for many years, is increasingly being seen as inadequate.
Bohr’s early work was carried out as a post-doc at Manchester University with Ernest Rutherford (10th in the list), whose famous experiments on the scattering of alpha particles from gold films showed that atoms have a nucleus. Rutherford’s work opened the door to the whole field of nuclear physics – and eventually to the development of nuclear energy and weapons by, among others, the Italian all-rounder Enrico Fermi (14th).
Marie Curie, who discovered the elements radium and polonium as well as making many other contributions in nuclear physics, comes 15th on the list with six votes. The only other woman to receive a vote was Cecilia Payne-Gaposhkin, the British-born astrophysicist who discovered that stars are amazingly uniform in their composition and that hydrogen is millions of times more abundant than any other element in the universe.
The most modern physicist in the top 15 is Richard Feynman (7th), who died in 1988 and who did much to develop our understanding of quantum electrodynamics – the quantum theory of the electromagnetic interaction. The final berth in the top 15 goes to Michael Faraday (joint 11th with Boltzmann), who in 1821 discovered that a wire carrying a current could be made to rotate in a magnetic field. His discovery paved the way for the development of both Maxwell’s theory of electromagnetism and the motor, which forms the basis of most of modern industry.
Of the 61 physicists voted for by respondents, 11 are still alive today. The nuclear physicist Hans Bethe leads this group with 3 points. But what about Einstein himself? Which physicists did he admire the most? According to the archives, his top three physicists were all British: Newton, Faraday and Maxwell.
The top physicists of all time
1 Albert Einstein |
2 Isaac Newton |
3 James Clerk Maxwell |
4 Niels Bohr |
5 Werner Heisenberg |
6 Galileo Galilei |
7 Richard Feynman |
8= Paul Dirac |
8= Erwin Schrödinger |
10 Ernest Rutherford |
11= Ludwig Boltzmann 1844-1906 Austrian, Michael Faraday 1791-1867 British, Max Planck 1858-1947 German: 16 votes each
14 Enrico Fermi 1901-1954 Italian: 13 votes 15 Marie Curie 1867-1934 Polish/French: 6 votes 16= John Bardeen 1908-1991 American, Lev Landau 1908-1968 Russian: 4 votes each 18= John Bell 1928-1990 British, Hans Bethe born 1906 German/American, Josiah Gibbs 1839-1903 American: 3 votes each. Two votes: Archimedes, Nicolas Copernicus, Pierre Curie, Gerard ‘t Hooft, Edwin Hubble, Johannes Kepler, Wolfgang Pauli, William Shockley, J J Thomson, Charles Townes, Steven Weinberg, Hideki Yukawa. One vote: Carl Anderson, Aristotle, Charles Bennett, Gerd Binnig, Felix Bloch, Nicolas Carnot, Rudolf Clausius, Democritos, Christian Doppler, Thomas Edison, Euclid, Arthur Eddington, Leonhard Euler, Stephen Hawking, David Hilbert, Paul Langevin, Hendrik Lorentz, Albert Michelson, Lars Onsager, Cecilia Payne-Gaposchkin, Lord Rayleigh, Martin Rees, Heinrich Rohrer, Wilhelm Röntgen, Arthur Schawlow, Alan Turing, John Wheeler, Kent Wilson, Chen Ning Yang. Names in bold indicate physicists who are still alive. |
Q3. What is the biggest unsolved problem in your field?
Q4. What is the biggest unsolved problem in the rest of physics?
Answers to our third question were obviously influenced by the make-up of our sample. However, they were remarkably similar to the answers to the fourth question, and both sets of responses were dominated by three broad topics: particle physics (including the unification of the forces, quantum gravity, theories of everything and so on), astrophysics (the big bang, dark matter, the cosmological constant), and the many mysteries of quantum mechanics. As Anton Zeilinger from the University of Vienna said: “In quantum physics the biggest unsolved problem is the question of what we actually describe in our theory. What is observation? What is information?”.
Daniel Greenberger of the City College of New York expanded on the difficulties in quantum theory. “I don’t think we understand the true significance of non-local correlations that travel faster than light but do not let us communicate faster than light,” he said.
There were other, clearly defined problems that cropped up regularly in both sets of answers, and which are explored in more detail in articles elsewhere in this issue. These challenges include climate change, fusion energy, the structure of the nucleus and solar magnetism.

Many respondents also cited problems in condensed-matter physics, with the origins of high-temperature superconductivity being undoubtedly the most common. Other problems included turbulence, melting, the glass transition and the structure of liquids. “Most problems in condensed-matter physics are unsolved, sometimes because they are too complicated, and sometimes because no one has seen the simplifying pattern,” explained David Thouless from the University of Washington in Seattle. “Obvious guesses about a big breakthrough in understanding glass formation or protein folding will probably be wrong. On the experimental side, the search for a room-temperature superconductor is an exciting challenge.”
Complexity was a common theme, notably the application of ideas from physics to progressively larger and more complicated structures – and ultimately to the brain and living organisms. “The new frontier about which we know nothing is how to describe complex systems far from equilibrium in a unified way,” said Peter Wolynes, a biophysicist at the University of Illinois. “Such systems range from sand piles to biological cells to computers, but it is not clear whether or how the principles of statistical mechanics apply to them.”
The challenge, said Giorgio Margaritondo from the Ecole Polytechnique Fédérale de Lausanne in Switzerland, was “to develop a general theory of complex systems, in particular of living systems, without relying on a ‘reductionist’ approach, which is based on the illusion that complex systems can be explained based on an understanding of their more elementary components”.
Edward Teller, commonly referred to as “the father of the H-bomb”, made a similar point: “Physics seems to have explanations that are somewhat complete for everything except life,” he said. “Does the explanation of life require an entirely new approach?”
As for atomic, molecular and optical physics, Wolfgang Ketterle of the Massachusetts Institute of Technology said that while these fields do not have a holy grail, there are nevertheless many important goals. These include developing practical atom lasers, advancing the precision of atomic-physics measurements even further, studying fundamental interactions (such as new forces or the violation of time-reversal symmetry), and controlling more complicated quantum-mechanical states. “Maybe after advancing to single atoms, single photons and single quantum states, atomic physics will turn back and try to achieve absolute control over more complex systems – quantum computation is one aspect of this,” said Ketterle.
And although many respondents emphasized (in their answers to our first question) the importance of the discovery that the universe could be understood by physical laws, deep questions about the nature of these laws still remain. “To what extent are the laws of physics unique?” asked particle theorist Frank Wilczek from the Institute for Advanced Study in Princeton, before going on to outline three possibilities. “The first option is that there is a unique fundamental equation with a unique stable solution. The second is a unique, fundamental equation that has many consistent solutions, with the particular solution that describes our world being picked out by some historical accident. Perhaps different, distant parts of the universe, not yet observed, experience different laws. Thirdly, there are a range of possible equations, each of which is equivalent for ‘practical’ purposes and for which the first two options apply.”
Brian Greene from Columbia continued this philosophical thread within string theory. “String theory is in need of a full formulation of its basic structure – its basic equations, so to speak – that does not rely on approximate methods. This formulation should be so general that the notions of space and time should emerge from the equations, as opposed to being ‘put in by hand’.” He added that such a formulation would take us a giant step closer to solving what he called “the biggest unsolved problem in string theory”, which is whether string theory can successfully predict the most fundamental properties of the matter and forces of our universe. “[Can string theory say] why are there electrons and other particles, and why do they have the properties (mass, charge and so on) that they do? And why do we observe four forces and why do they have the properties they do?”
Greene went on to point out that the universe as we know it depends on delicate relationships between the properties of particles and forces. “If the strengths of the forces or masses of the particles were different by even a few per cent, stars would not ignite and the universe would be very, very different place. Can string theory explain the particle and force properties? That is, can it explain why the universe is the way it is?”
Wilczek ended with a word of warning. “I do not like this question because it encourages a dangerous tendency,” he said. “In science, while we should keep the ‘greatest’ unsolved problems in mind, in practice we have to balance the intrinsic grandeur of problems with our ability to solve them. This is a very basic point, which is often obscured or lost in popular treatments of science.”
Wilczek pointed to Galileo as an example. “He was surrounded by great professors of theology, Aristotelian philosophy, and so forth, who addressed all the ‘greatest’ questions about the nature of the universe, the meaning of life, and so on, in long treatises. But Galileo made a more lasting contribution by studying and figuring out precisely how balls roll down inclined planes.”
Q5. Would you study physics if you were starting university this year?
The vast majority of the physicists we contacted are happy with their subject. Some 70% of respondents said they would study physics if they were starting university this year, while 13% were undecided or not sure. Only 17% said they would not choose physics – and that included some who answered on the basis that they were being given a second life and that one lifetime in physics was enough. As one Japanese physicist put it: “I worked too hard. I want to enjoy life next time.”
However, the bald statistics do not tell the whole story, and the answers to this question encapsulate much that is good and bad about physics at the turn of the millennium. Jorgen Kjems, director of the Risø National Laboratory in Denmark, summed up the opinion of many: “I do not know of any other discipline that offers a similarly rich combination of mathematical rigour, room for imaginative creativity and rewarding interplay between experiment and theory.” Others called physics “the most grandiose science”, “the most fascinating activity for our brain”, “still the most fundamental of all sciences” and “the best training if you want to enter another field”.
But the responses of those who would not study physics again reveal a darker side to the subject. Martin Rees, the UK’s Astronomer Royal, said he would be “unlikely to be inspired by [today’s] typical student physics curriculum”, while others grumbled about poor pay, inadequate career structures and a lack of decent physics teachers.
The increasing stress placed on the “relevance” of basic research was also cited by several respondents. “The constraints imposed on the physicist today by government funding make the field far less attractive,” sighed astrophysicist Eugene Parker from the University of Chicago. “The idea that the relevance of projects in pure research must be evaluated before the research has been carried out threatens to be stifling.” Nevertheless, Parker would still chose to do physics if given the chance again.
Many respondents complained that physics is now simply too large. “Research activities have become too big, and the lead-in times are too long for young starters to make an impact,” warned John Eades from CERN.
Others said it was too hard to produce radical new results, with a lack of major breakthroughs on the horizon. “I have the feeling that physics is approaching a point of diminishing return; even if new results are constantly emerging, they are more costly and less fundamental in most cases,” said laser physicist Stig Stenholm from the Royal Institute of Technology in Stockholm.
Even Gerard ‘t Hooft from the University of Utrecht, who shared the 1999 Nobel prize for his theoretical contributions to the Standard Model, had his doubts about signing up for physics again. “I would do physics, but I am not sure whether it would be the right decision,” he said. “Physics is entering a phase where it is getting increasingly difficult for young people to produce interesting and important new results. Instead, the sociology of bluffing one’s peers about the purported importance of one’s results is becoming more important than it should be.”
Many respondents who answered “no” or “maybe” were tempted by the biosciences. As Michael Green, a particle theorist at Cambridge University, pointed out: “There is something attractive about a subject that is still in a relatively primitive state.”
That view was shared in industry too. “Physics is no longer one of the most exciting fields of research in which major new discoveries will be made,” said Charles Duke, vice-president for research at Xerox. “More attractive opportunities exist in molecular biology and information-systems research.” Duke would not study physics second time round.
Others saw computing as the better option. “On the whole, physics is boring,” complained Artur Ekert of Oxford University. “Information processing both by computers and biological systems sounds more attractive these days.” Edward Teller would also go into computing because “the application of new computing capabilities to science is apt to produce remarkable results”.
But the problem for most physicists is that they are in love with their subject. Michio Kaku expressed it best: “Even if job prospects are not very good, we physicists would rather starve than leave the field. I meet so many people today who say that now that they have a comfortable middle-class life, they deeply regret not sticking to physics when they were in college. Their decision to leave science will haunt them forever.”
Q6. If you were starting your research career in physics again, which areas of physics would you go into?
“It is important that students pay particular attention to two criteria when choosing a research field: having fun, and matching their talents and skills with the work required in that field.” That is the advice from astrophysicist John Bahcall from the Institute for Advanced Study in Princeton. And those who replied to our survey appear to have followed his advice because most seem happy with their careers to date. Indeed, the number of respondents who would choose the same field of physics again out-numbered those who would try something else by a factor of more than two to one.

It is hard to be categorical, but astronomers and astrophysicists appeared keenest to remain in the same field. “The study of the universe will become the major theme in physics in the 21st century,” predicted Nikos Prantzos of the Institut d’Astrophysique in Paris. “Astrophysics is where the action is today,” added Lincoln Wolfenstein of Carnegie Mellon University in the US. “There are new discoveries and new problems every year.”
Even Martin Rees, who had said he was underwhelmed by today’s undergraduate physics curricula, would remain in the field: “Astrophysics and cosmology have the highest ratio of problems to people, and an impressive rate of discovery at the present time.” Astrophysics and cosmology also appealed to many physicists outside the field.
However, many respondents would prefer to move into the biological sciences because they felt that they could make serious contributions more quickly and more easily in this field than in physics. “The central disciplines of physics are crowded with too many people chasing too few ideas,” explained David Thouless. “I would opt for physics applied to some biological subject, where the fashionable ideas are remote from physics.”
Andre Geim from the University of Nijmegen in the Netherlands selected biophysics or genetics, where, as he put it, “a physicist can really do something in an area where mostly craftsmen work”. Ian Aitchison, a theorist at Oxford University, would also choose biology: “The pace there is far quicker, new methods are coming in fast, and the chance of making a serious contribution is higher.” Others saw the interface of physics, biology and computing as one of the most promising areas for the 21st century.
But many physicists spoke up eloquently in praise of their own field. “I cannot imagine looking at anything more awe-inspiring than atomic arrangements in materials, or anything so easy to justify as contributing to the economy,” enthused Steve Pennycook, an electron microscopist from the Oak Ridge National Laboratory in the US. “I would certainly go into statistical physics again,” said Constantino Tsallis of the Brazilian Center for Research in Physics. “Its enormous complexity and richness make it irresistibly fascinating.”
John Houghton, who is co-chairman for science assessment on the Intergovernmental Panel on Climate Change, extolled the virtues of environmental physics. The field was, he said, “theoretically and experimentally extremely challenging. It also involves exciting areas of technology, science and computing, and is highly relevant to human society and its future”.
Chris Quigg, head of particle theory at Fermilab, praised high-energy physics. “I do think that the best days of particle physics lie ahead, and I wouldn’t hesitate to encourage a student – even a young me – to join in.”
Others had unusual reasons for choosing particular subjects. David Mermin from Cornell University, for example, chose quantum information theory because “it has some very interesting people working in it, and because one can always bail out into conventional computer science when it becomes obvious that no functioning quantum computer can be built”.
But the most honest assessment came from John Ziman, emeritus professor at Bristol University in the UK. “[I would choose] whatever area first captured my interest and attention, because once one gets into them, all fields of research are equally laborious and tedious, and all fields of research are equally fascinating and exhilarating.” Who could argue with that?
Q7. Stephen Hawking has said that there is a 50-50 chance that we will find a complete unified theory in the next 20 years. Do you agree that the end of theoretical physics is in sight?
Our final question was slightly different to the previous six, but the answer was a resounding no! Although our question was deliberately provocative – as Hawking’s friend Kip Thorne replied: “I cannot help but remark that your last question is outrageously worded” – several respondents noted that Hawking had made this statement at various stages in his career, including his inaugural lecture as the Lucasian professor of mathematics at Cambridge in 1980 and at various public lectures during the 1990s. Unfortunately Hawking himself declined to take part in our survey.
The most common criticism was equating the discovery of a theory that unified the four fundamental forces of nature – a so-called theory of everything – with the end of theoretical physics. Some theoretical particle physicists agreed with Hawking’s prediction about the chances of discovering a theory of everything, although several reckoned that it would take 50 to 100 years. Steven Weinberg, for example, said: “20 years is possible, but unlikely. I would guess 100 years for a ‘complete unified theory’. But a ‘complete unified theory’ would not be the end of theoretical physics.”

Tom Kibble from Imperial College, London, and Michio Kaku made similar points. “I agree that there is a 50-50 chance that we will find a complete unified field theory describing all the fundamental forces and particles within the next 20 years,” said Kibble. “[But] I strongly disagree with the idea that this will mean the end of theoretical physics – this is a reductionist myth. Knowing the basic laws – as we apparently do in condensed-matter physics, for example – certainly does not mean the end of surprising discoveries and exciting new theoretical developments. I am very confident that there will still be many exciting things for theoretical physicists to study.”
Kaku agreed that 20 years will be enough “to prove whether superstring theory is the theory of everything or the theory of nothing – there is no middle path. But even then, knowing the rules of chess does not mean we have become grand masters of chess. Similarly, knowing the rules of the unified field theory does not mean we have become grand masters of that theory. It may take us centuries before we exhaust the full implications and applications of a theory of everything”.
Gerard ‘t Hooft, however, was less optimistic about even the more limited interpretation of Hawking’s statement: “Absolutely not. He has been saying the same thing for more than 20 years. Physicists like him will say this again and again, always projecting the ultimate solution 20 years to the future. Although I do believe an ultimate theory is conceivable, we are many generations away from it.”
“Physics is not like getting to the top of Everest,” said Luciano Maiani, director general of CERN. “It is more like trying to get to absolute-zero temperature. As you get closer, new scales of phenomena appear and these call for a new effort and new understanding.” Current experiments in particle physics probe the Fermi scale – at energies of about 200 times the proton mass – and we know, said Maiani, that new phenomena – such as, perhaps, supersymmetry – must appear at an energy that may be about ten times larger. Quantum gravity, on the other hand, will not be testable until we reach the Planck scale, which is about 1019 times the proton mass. “Hawking’s ‘prediction’ is based on the idea that nothing happens in between,” said Maiani, “and that everything will follow if we can unite gravity to quantum theory, with a purely theoretical effort. I think this scenario is far from being proved and even far from being convincing.”
Eugene Parker at Chicago was not convinced either. “The idea that when the last field equation is written down on paper, physics will come to an end is naive in the extreme,” he said. “In 1865, for example, Maxwell completed the electromagnetic field equations by adding the displacement current to Ampère’s law. That was the beginning of electromagnetism, not the end. When Schrödinger and then Dirac wrote down the quantum-mechanical wave equation, that was the beginning of quantum mechanics, not the end. When Einstein wrote down the equations of general relativity, that was the beginning of modern gravitational theory and cosmology, not the end. You get the idea.”
Many respondents pointed out that the discovery of a theory of everything will have little impact on the rest of physics “A unified theory would be a tremendous breakthrough,” said astronomer Alex Filipenko at the University of California at Berkeley, “but it would not, for example, lead to solutions of many important problems in condensed-matter physics, biophysics, astrophysics, and so on. It certainly won’t give us a much clearer picture of the origin of life or of intelligence. Much will remain to be done!”
Princeton University’s Phil Anderson was more direct: “The question is an insult to me and to all those who call themselves theoretical physicists. A unified theory is unlikely to tell us much at all, though it may simplify a few questions about cosmology. Theoretical physics has plenty of problems on the frontier of complexity to keep us busy for quite a while. For instance, the problem of how life originated is at least partially a physics one.”
Jean Zinn-Justin, a theorist at the Saclay laboratory of the French atomic energy commission (CEA), continued in this vein. “Many major discoveries have happened in areas where the basic interactions were already well known,” said Zinn-Justin. The challenge, he added, is to understand what comes out of the interactions between many elementary constituents. “Nobody claims that the study of the brain reduces to understanding the connections between neurones and the chemistry involved in brain activity. It is possible to devise unending new systems with only nuclei, electrons and photons, so there will probably be interesting theoretical physics, as long as there are human beings around.”
“Reductionism has failed in a grandiose manner,” said Itamar Procaccia from the Weizmann Institute of Science in Israel. “To understand macroscopic phenomena, which are all around us, we cannot start from strings. Every level of description has its own logic, mathematics and phenomenology. A tremendous lot remains to be done even if a unified theory is achieved.”
New data and observations will also play a crucial role. “One simply cannot predict where the major advances will occur,” said Peter McClintock, a low-temperature physicist at Lancaster University in the UK. “But they will probably arise where experimentalists manage to falsify accepted theories.”
Astrophysicist Vera Rubin from the Carnegie Institute in Washington, DC, made a similar point. “Even if we believe in 20 years that all of physics is then known, I believe that later discoveries and astronomical observations will show that we were wrong. But that, of course, is the beauty of science. It is hard to believe that 500 years from now, some of our concepts will not be put in the ‘brilliant, but primitive’ class.”
A handful of respondents resorted to humour. “NO!” said Cornell’s David Mermin. “My guess is that more and more structure will be found at shorter and shorter length scales, until it becomes boring even to the particle physicists.” Chris Quigg of Fermilab obviously wanted the last word: “I hope that the end of foolish pronouncements by theoretical physicists is in sight!”