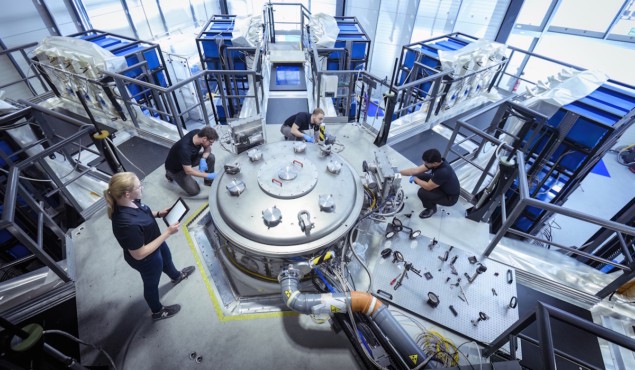
Harnessing the power of nuclear fusion to generate electricity is a longstanding aspiration of the physics community. One company working towards this goal is UK-based First Light Fusion, which is using a technique called projectile fusion to create a simple, low-cost inertial fusion power plant.
Having confirmed last year that its technology can achieve fusion, First Light is now developing a net-energy-gain demonstrator, known as Machine 4. And while working with Spanish engineering firm IDOM to optimize the reactor design, the team realized that projectile fusion could also be used to produce a variety of sought-after medical isotopes.
Inertial confinement fusion, one of two main fusion technologies under development, works by compressing a small fuel target containing a mixture of hydrogen isotopes. At high enough temperatures and pressures, nuclear fusion reactions occur between deuterium and tritium in the target.
The most common inertial fusion technique – and the approach with which the National Ignition Facility first demonstrated energy gain from fusion last December – uses high-powered lasers to trigger the fusion. First Light is developing a different approach, in which a high-velocity (and low cost) projectile is fired at the target. An amplifier within the target focuses the projectile’s energy, with the resulting shock waves squeezing the fuel so much that it gets hot and dense enough to fuse and release a pulse of energy.
The majority of the energy released by fusion is in the form of high-energy neutrons. This energy is absorbed by a blanket of liquid lithium lining the reactor’s inner wall, and the heat can be extracted to generate electricity. The neutrons are also used to create tritium, via a reaction with the liquid lithium blanket.
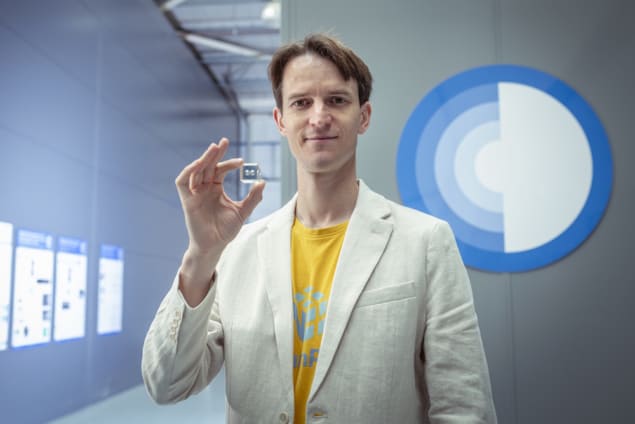
“While the main focus of the reactor was originally the production of electricity from fusion, the First Light reactor also produces tritium to be self-sufficient in tritium fuel,” explains Nick Hawker, First Light’s co-founder and CEO. “Tritium is used by most other fusion reactors and is in short supply, so we started to vary the design to see if over-production of tritium was possible. During these investigations, Hawker explains, “we also realized that these neutrons could be useful for isotope production”.
In-demand isotopes
Radioisotopes are widely employed within medicine for a range of diagnostic and therapeutic applications. The most prevalent is technetium-99m (Tc-99m), the decay product of molybdenum-99 (Mo-99). Tc-99m is employed in tens of millions of nuclear medicine procedures each year, including myocardial perfusion imaging to diagnose heart disease, and scans to detect and stage cancer.
At present, Mo-99 is typically made using fission-based production in a few ageing nuclear reactors and, due to a half-life of just a few days, it can’t be stored. As such, there’s a growing demand for new ways to produce Mo-99 and other medical isotopes. One approach is to bombard nuclei of stable elements with high-energy neutrons – such as those produced by First Light’s reactor – causing them to transmute into the required radioisotope.
“The neutrons produced by fusion are high energy, and the flux is also very high. This means that there is a very wide scope for isotope production with fusion as the neutron source,” Hawker explains. “We can in theory create a large range of different isotopes, including Mo-99.” The team has also studied isotopes used for cancer treatments, including copper-67, samarium-153, lutetium-177 and yttrium-90.
Neutron-induced reactions are energy dependant, with some transmutations necessitating high-energy neutrons. As First Light’s reactor will produce high-energy 14 MeV neutrons, the company may benefit from creating isotopes that have a threshold reaction energy for production. “While most isotopes can be made with high-energy neutron bombardment, we must find where we are cost competitive or have a unique advantage,” Hawker tells Physics World.
Hawker notes that First Light originally discounted the idea of isotope production as the reactor’s liquid blanket makes it difficult to place a sample on the first wall, where the neutron flux is highest. However, the team has now designed a way to control neutron paths through the blanket, allowing neutrons to penetrate and focus on specific regions where isotope production components can be placed.
Importantly, this can be achieved without compromising the reactor’s ability to produce electricity. “We can have a thick blanket for good tritium and electrical production, but also allow neutrons out in a focused region (which can move) for isotope production,” Hawker explains. “This means that we can address all three opportunities with little compromise.”
First Light continues to work closely with IDOM on the design of its reactor chamber and the development of Machine 4, which will be housed in a purpose-built facility at the UK Atomic Energy Authority’s Culham Campus in Oxfordshire. Construction is anticipated to begin next year, with operations likely to commence in 2027. “Once the system is performing high-yield shots, this may give us an opportunity to test the isotope production as a proof of principle,” says Hawker.
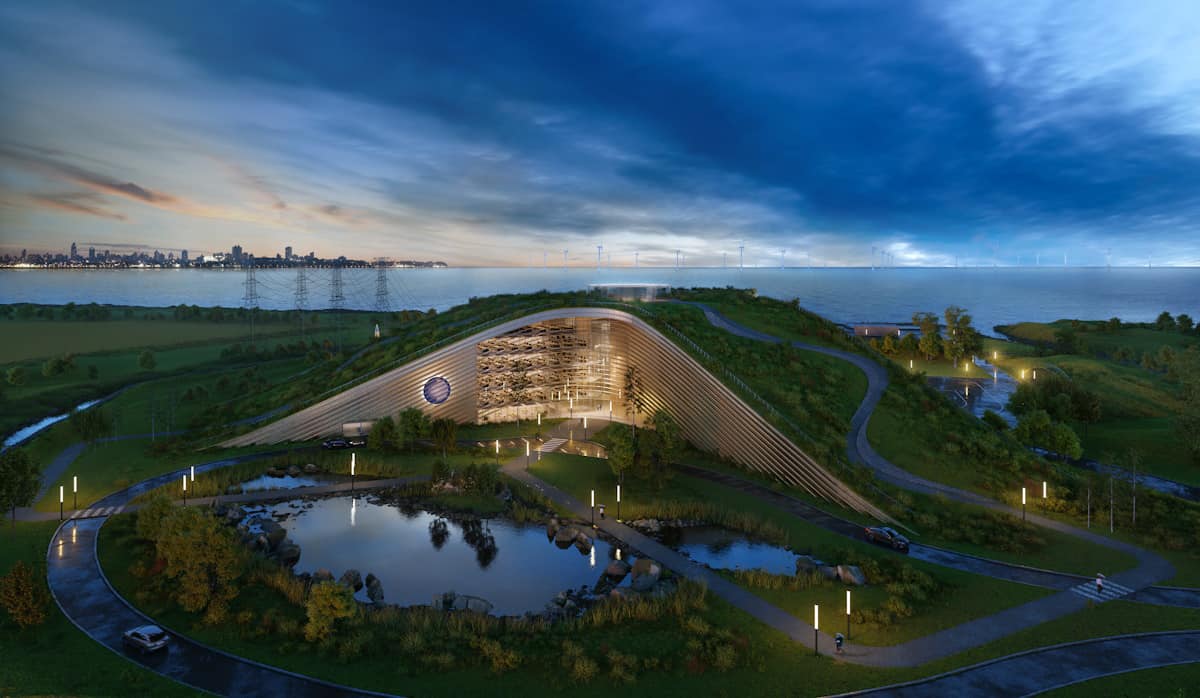
Proven approach
First Light is not the only company exploiting fusion to create medical isotopes. SHINE Technologies of the US is using its fusion technology to produce the therapeutic isotope lutetium-177 (Lu-177). SHINE made its first commercial sales of Lu-177 in 2020 and recently opened the largest Lu-177 production facility in North America at its headquarters in Janesville, WI. The company is also currently constructing a fusion-driven medical isotope production facility, the Chrysalis, to produce Mo-99.
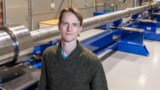
Projectile fusion offers new path to clean energy, quantum communications for alien civilizations
Medical isotope production is Phase 2 of SHINE’s four-phase roadmap towards generating fusion energy. “Advancements in radiopharmaceutical therapies have shown great results in extending the lives of patients who might otherwise be out of options,” says SHINE’s founder and CEO Greg Piefer. “We’re excited to play an important role in making sure these groundbreaking therapies reach patients faster, potentially saving or extending the lives of many tens of thousands of people each year.”
“We believe that fusion has the potential to produce isotopes to diagnose and treat cancer,” Piefer tells Physics World. “We see ourselves as pioneers of that vision, and it’s great to see other companies that are interested in developing solutions that reduce the market dependency on reactors as well.”