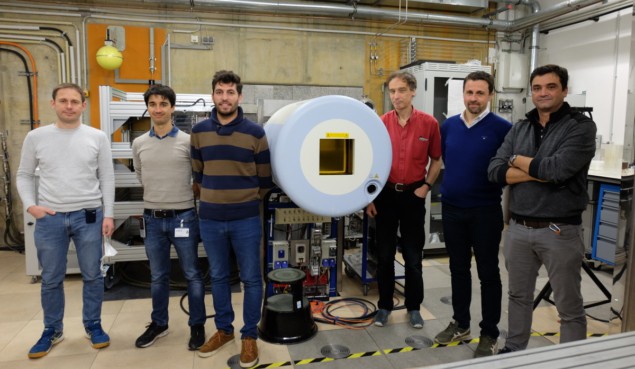
Prompt gamma spectroscopy (PGS) is a promising technique for monitoring the range of protons delivered during proton therapy. Its ability to measure absolute range deviations during a course of treatment and provide real-time feedback could allow for immediate changes if organ movement is identified during treatment. For proton therapy of prostate cancer, the use of PGS to monitor rectal radiation exposure in real time could potentially reduce the risk of gastrointestinal toxicities.
Proton therapy is designed to target the most difficult-to-treat and hard-to-reach tumours, and enables higher doses of radiation to be delivered to the prostate using proton beams of less than a millimetre in diameter. Because the energy from a proton beam can damage healthy tissue near the targeted tumour, real-time monitoring is of critical importance to verify the precision of dose delivery. In the case of prostate cancer treatments, for example, high radiation dose to the rectum correlates with increased gastrointestinal toxicity.
A multinational research team coordinated by Joao Seco of the German Cancer Research Center (DKFZ), is investigating the use of PGS to monitor rectal radiation exposure during prostate cancer proton therapy, using an endorectal balloon (employed to stabilize prostate location during treatment) inflated with a silicon dioxide/water mixture.
Seco, together with first author Paulo Magalhaes Martins and Hugo Freitas at DKFZ, and Stephan Brons, Benjamin Ackermann and Thomas Tessonnier from the Heidelberg Ion-Beam Therapy Center (HIT), investigated PGS with both water-filled and silicon dioxide/water-filled balloons inserted into a prostate phantom. They report their findings in Scientific Reports.
Silicon as a range probe
PGS works by analysing the energy spectrum of prompt gamma rays emitted when charged particles such as protons irradiate atomic nuclei within the human body. These gamma rays have characteristic energy lines that reflect the elemental composition of the irradiated tissue. The researchers determined that protons hitting silicon atoms in the endorectal balloon emit prompt gamma rays with a unique energy of 1.78 MeV, which is distinguishable from the tissue spectrum. This finding suggests that the use of a silicon dioxide/water-filled balloon could serve as a real-time range verification probe during a standard 2 Gy proton therapy fraction.
The team initially irradiated various water solutions and mixtures with single-spot proton beams, increasing the beam energy to a level applicable for prostate cancer treatment. Based on their findings and its lack of toxic effects, the researchers continued their investigations with a mixture of water and silicon dioxide (from diatomaceous earth).

Next, the researchers irradiated a prostate phantom containing the silicon dioxide/water-filled balloon with horizontal single-spot proton beams at different positions. To replicate a clinical treatment scenario with a rotating gantry, they rotated the phantom by 90° in the transaxial direction at gantry angles of 0°, 90° and 270°. To monitor the ion-beam-induced prompt gamma rays, they used cerium bromide scintillation detectors, which measure the entire prompt gamma emission spectrum.
The team also delivered treatment-like plans to the phantom, with an anterior beam irradiating the prostate either conformally or overlapping with the endorectal balloon. The conformal plans were designed to deliver a maximum rectal dose below 0.3 Gy per 2 Gy fraction, and to prevent any Bragg peak localization within the balloon. The overlapping plans covered an extended target including the prostate and a 1.5 cm extension towards the balloon.
The researchers demonstrated that PGS could identify iso-energy layers crossing the balloon, as well as the columns parallel to the balloon within each iso-energy layer. They also validated the same method using an anterior–oblique field with a gantry angle of 279°. Such real-time feedback would allow clinicians to decide whether to adapt or continue treatment.
Sharing the data
In a related manuscript published in Scientific Data, the team subsequently developed a PGS dataset obtained after irradiating the prostate phantom with the inserted endorectal balloon (filled either with water or a silicon dioxide/water mixture) with 43 single-spot anterior beams of defined proton energies. The data from these measurements, and those described above, provided enough evidence to determine the presence of the silicon in the beam path above a certain beam energy.
“Such evidence is crucial to monitor the irradiation of the rectal wall in anterior beams and may open new possibilities for future control or prevention,” the researchers write. “The energies used are within the range of energies available in most proton centres either with passive scattering or active scanning delivery.”

Prompt gammas could measure body composition during particle therapy
In both measurement campaigns, the researchers irradiated different regions of the phantom with single spots, increasing the energy of the proton beam in sequential steps from 86.72 to 134.06 MeV and obtaining a prompt gamma energy spectrum for every beam energy.
The researchers have made the dataset available to researchers in the figshare repository. They hope that the dataset will provide other researchers with the tools to reproduce their results and evaluate alternative geometries, beam species and phantoms.