
Protons. Destroying cancer cells more precisely than X-rays. Depositing less dose in healthy tissues. Verifying treatment plans and improving patient alignment?
Christina Sarosiek, a graduate student at Northern Illinois University, is working on that.
“The goal of our project is to make proton therapy safer and more effective using an imaging modality called proton radiography,” she explains. “We’re able to take a picture of the tumour directly before treatment and therefore know that we’re irradiating the tumour and not healthy tissues.”
Creating radiographs with protons
Like all medical therapies, proton therapy has some uncertainties. Changes in patient anatomy between treatments, small misalignments of the patient, or errors in the calibration from a planning CT scan to a proton treatment plan, for example, can all lead to underdosing a tumour or delivering dose to healthy tissues, neither of which are optimal.
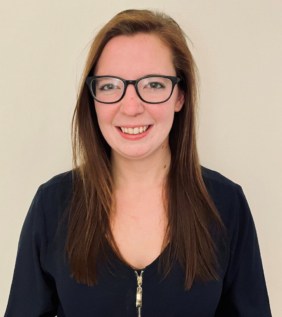
Sarosiek is part of an interdisciplinary team that is developing and characterizing a prototype proton radiography system that not only improves upon methods used today, but also may help scientists and clinicians tackle all of these other challenges.
For example, medical physicists can verify proton range in vivo using a range probe, which works by passing a low-dose, high-energy proton pencil beam (a very thin beam) through a patient and comparing the measured integral Bragg peak with that from a planning CT. But a range probe is limited because it doesn’t provide any spatial information and can’t improve patient alignment.
Proton radiography, on the other hand, works by sending very high-energy but low-intensity protons through a patient and then reconstructing an image based on the resulting data, which represents, pixel-by-pixel, the water-equivalent thickness – basically, how far a proton would have travelled if it were in water. The source of image contrast in a proton radiograph is the energy loss of the transmitted protons (the integrated stopping powers of protons in the patient).
“We’re getting an image of the integrated energy through an entire patient,” Sarosiek says. “If the anatomy changes or the [electron] density changes on the way to the tumour, we would see that appear as a difference in the full integration through the patient.”
To put this another way, a proton radiograph would tell a medical physicist or clinician if, but not precisely where, there was a difference from what was planned.
“Proton radiographs can alert us to range discrepancies in the plane perpendicular to the beam, but a single proton radiograph cannot inform us about exactly where along the beam path (that is, proximal or distal to the tumour) that discrepancy lies,” Sarosiek explains.
Image quality sufficient for pre-treatment range verification
Sarosiek and the team characterized their prototype proton radiography system using several different phantoms. They published the results of these studies in Medical Physics.
The proton radiography system and the team’s reconstruction algorithm produced images with high enough spatial resolution and image quality to help align a patient better right before their proton treatment starts.
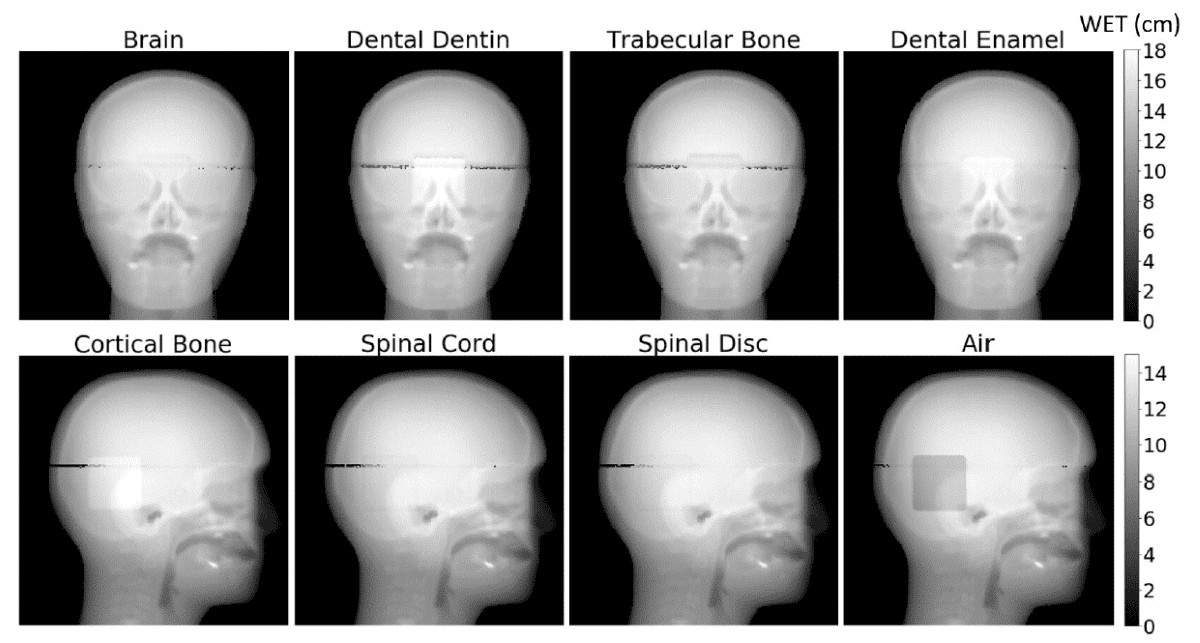
Results also illustrated that the system could be used to help clinicians with quality assurance, by detecting errors in treatment plans resulting from changes in patient density between a treatment planning CT and the proton treatment plan. These applications might ultimately help clinicians and medical physicists reduce margins in treatment planning.
Sarosiek says that their results are comparable to those in other studies, which rely on custom proton radiograph systems. One advantage of the system studied by Sarosiek and the rest of the team is that it is currently being optimized and commercialized by an industry collaborator.
An integrated proton imaging and treatment delivery system
“In the short term, we know [our proton radiography system] works. The long-term impact, I think, is that we may be able to use the same modality for imaging and therapy,” Sarosiek says.
Currently, proton therapy treatments are planned using an X-ray-based CT scan. CT images are displayed in Hounsfield units, which represent a transformation of the X-rays’ attenuation coefficients. Protons interact with tissue and deposit dose differently, and this presents an additional source of error for proton therapy.
Researchers are also working on limiting these errors. Several proton radiographs may be used to create patient-specific curves comparing CT dose to relative proton stopping power (RSP). The downside, though, is that thus far, studies looking into this are based on simulated data, Sarosiek says.
Another avenue being pursued is proton CT. Proton CT would allow medical physicists and clinicians to create a treatment plan directly using the proton CT and avoid a calibration curve like the CT-to-RSP curve examined by other groups. Sarosiek’s collaborators will be investigating this in the future.
For now, though, Sarosiek and the rest of the research team are focused on one of the immediate limitations of proton radiography before it can transition into clinical use.
“One of the major limitations [of this approach] is that for proton radiography, we require a high-energy, low-intensity proton beam. But clinical proton treatment beams have much higher intensity with lower energy,” Sarosiek says. That means that any integrated proton imaging and treatment delivery system would need onboard beam monitoring systems that analyse low-intensity imaging beams, ensuring that dose to the patient remains low.
Once this problem is solved, proton radiography can enter clinical practice, she says.