
Interactions between matter and light in microcavities made of mirrors are fundamentally important for many modern technologies, including lasers. Researchers at the University of Michigan, Ann Arbor, US, have now gained tighter control of these interactions by exploiting a nonlinear effect that occurs in a new kind of hybrid semiconductor made from bilayers of two-dimensional materials. These semiconducting sheets form an egg-carton-like array in which the “pockets” are quantum dots that can be controlled using light, and they could be used to make ultralow-energy switches.
Led by Hui Deng, the researchers made their hybrid semiconductor from flakes of tungsten disulphide (WS2) and molybdenum diselenide (MoSe2) just a few atoms thick. In their bulk form, these transition-metal dichalcogenides (TMDCs) act as indirect band-gap semiconductors. When scaled down to a monolayer thickness, however, they behave as direct band-gap semiconductors, capable of efficiently absorbing and emitting light.
When laid on top of one another, the electronic structures of TMDCs can form a larger electron lattice (known as a moiré lattice) thanks to the slight mismatch of the materials’ lattice constants. The period of this lattice can be tuned by twisting the monolayers with respect to each other at different angles. In the WS2 and MoSe2 bilayer studied in this work, this angle is about 56.5° and the moiré lattice produced contains “pockets” measuring around 10 atoms across. These pockets, explains study lead author Long Zhang, are the quantum dots – tiny pieces of semiconducting materials that can isolate individual quantum particles such as electrons.
Quantum dots confine excitons
In Deng and colleagues’ experiments, the “particles” thus isolated are excitons: particle-like excitations (quasiparticles) created when an electron in a semiconductor’s valence band is excited by a photon to the conduction band. A positively charged “hole” is then left behind in the valence band in the place of the electron. Because the electron remains strongly attracted to the hole, the two “pair up” and behave like a single entity – the exciton.
In conventional, linear, devices, excitons can travel freely throughout a device, so they hardly interact with each other. If the exciton is confined to a quantum dot, however, as in this new work, it is impossible to add a second identical exciton to the same quantum dot, explains Deng. To do this, a higher energy photon is required. “This is known as quantum blockade and causes the nonlinearity we have seen in our experiments,” she adds.
Since quantum dots are only a few atoms across, they are too small for practical applications. Deng and colleagues therefore created an array of quantum dots that they describe as contributing to the nonlinearity “all at once”.
Mirrored microcavity
To control the arrays of dots as a group using light inside the 2D semiconductors, the researchers built a resonator by embedding the 2D hybrid semiconductor between two mirrors that form a microcavity. When they excited the structure with red laser light, they found that it resonated within the cavity and formed another quasiparticle, called a polariton, which is a hybrid of an exciton and light. This observation, they explain, confirms that all the quantum dots are interacting with light in concert.
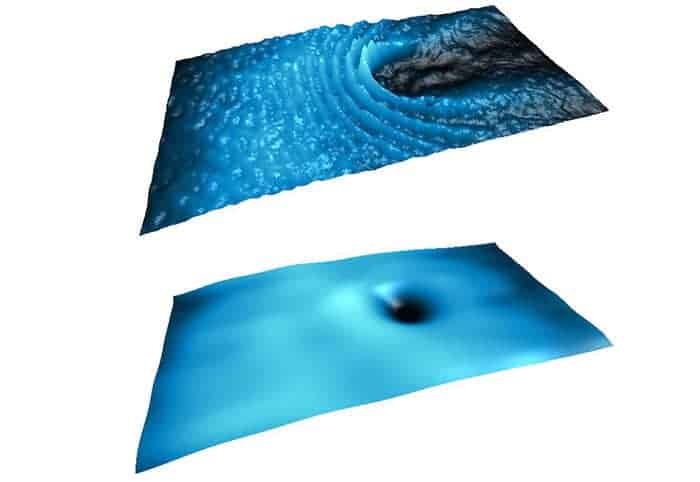
Superfluid polaritons seen at room temperature
When the researchers then introduced a few excitons into the material lattice, they observed a measurable change of the polariton’s energy. This implies that the system is showing nonlinear behaviour due to quantum blockade, Deng says.
“Engineers can use that nonlinearity to discern the energy deposited into the system, potentially down to that of a single photon, which makes the system promising as an ultralow-energy switch,” she explains.
The researchers say their work, which they report in Nature, might be extended to achieve polariton blockade similar to the exciton blockade seen in their experiments. “We also plan to increase the nonlinearity we have observed by varying the moiré lattice and reducing the size of the cavity, and look for ways to create quantum states of light from the system,” Deng tells Physics World.