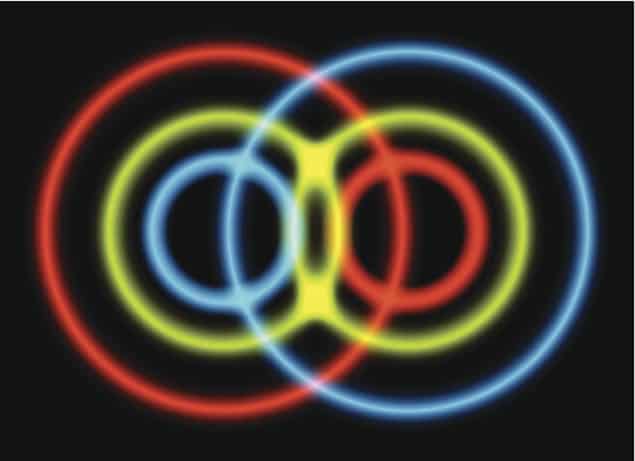
The elusive, yet seemingly inevitable, quantum nature of gravity may finally be demonstrated experimentally. Two separate groups in the UK; Chiara Marletto and Vlatko Vedral at the University of Oxford, and a team led by Sougato Bose from University College London, have proposed experiments that may for the first time reveal a link between the theories of quantum mechanics and general relativity.
Within their domains, both quantum mechanics and Einstein’s general theory of relativity seem to work incredibly well, no matter what problems physicists throw at them. However, some of the physical rules overseeing the theories appear to be fundamentally incompatible, and a unified theory of quantum gravity which marries them together has become notoriously difficult to develop.
“A formidable problem is the immense weakness of the gravitational interaction in comparison to other fundamental forces in nature,” Bose tells Physics World. “For example, even the electrostatic force between two electrons overtakes the gravitational force between two kilogram masses by several orders of magnitude.”
Impossible to test
So far, theories about the quantum nature of gravity have seemed practically impossible to test experimentally. As such, theories ranging from quantum particles known as gravitons, which convey gravity in a similar way to photons for electromagnetic fields, to the all-encompassing ideas of string theory and loop quantum gravity, have all remained speculative.
Early steps to devise an experiment to test quantum gravity were taken by Richard Feynman. He proposed a thought experiment in which a test mass is prepared in a quantum superposition of two different locations, and then interacted with the gravitational field, causing the mass and the field to become entangled. If the two spatial states of the mass could then interfere; reverting the mass back to a single, definite spatial location, the coupling with the gravitational field would subsequently be reversed, showing that gravity has been coherently coupled to a quantum system.
Feynman hoped that this experiment would confirm the quantum nature of gravitational fields, but Marletto and Vedral believe it is not sophisticated enough. Since the interference of the two spatial states of the mass could occur even in the presence of classical gravitational fields, the experiment would not prove that the mass and the field were entangled, unless the entanglement could be measured directly. Therefore, the gravitational field need not be quantized. Bose explains, “Exactly what attribute of the masses to measure in order to conclude the quantum nature of the gravitational field was left open”. Another approach was needed to witness its quantum features.
Entanglement between two masses
Bose, Marletto and their colleagues believe their proposals constitute an improvement on Feynman’s idea. They are based on testing whether the mass could be entangled with a second identical mass via the gravitational field. To do this, the two masses would first be prepared using two adjacent, identical interferometers. These devices are typically used to split light waves into separate beams, which can then be interfered. When tiny masses are involved, however, their quantum wave functions can be split and interfered to superimpose multiple quantum states onto the mass.
“Our two teams took slightly different approaches to the proposal,” Marletto explains to Physics World. “Vedral and I provided a general proof of the fact that any system that can mediate entanglement between two quantum systems must itself be quantum. On the other hand, Bose and his team discussed the details of a specific experiment, using two spin states to create the spatial superposition of the masses.”
If gravitational fields are truly quantum in nature, both teams realized the gravitational attraction between the two masses would cause them to become entangled by the time they left their respective interferometers. As in Feynman’s experiment, the first mass can be entangled with the gravitational field, but this time, no interaction with the gravitational field is required since the second mass could be used as a witness to the quantum properties of the first. This would allow the researchers to confirm that classical gravitational fields could not have been responsible for the interference of the masses.
Unwanted effects
Bose and Marletto’s teams both acknowledge that the challenges posed by shortcomings in present-day technology mean that their proposed experiments have no guarantee of success. If the experiments are not carried out carefully enough, other stronger forces like the Casimir, Van der Waal’s or other unwanted electromagnetic interactions could mimic the desired effects of gravity, entangling the two masses.
The masses could also fail to become entangled even if the gravitational field is quantized, should the nature of quantum gravity be even more subtle and complex than the researchers anticipated, meaning not witnessing entanglement would not be an inconclusive result. They also realize that their experiments still wouldn’t confirm which of the many competing theories of quantum gravity are correct.
The proposals are described in two papers published in Physical Review Letters: C Marletto and V Vedral and Bose et al.