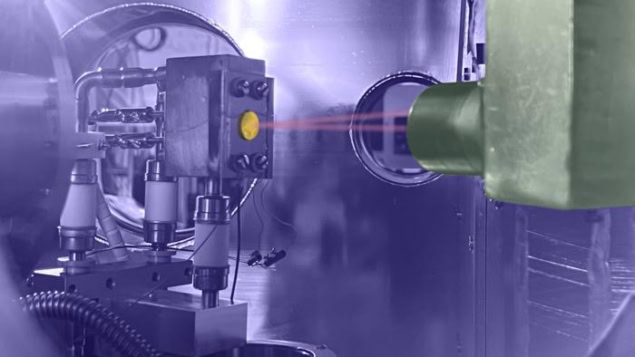
A team of researchers in Switzerland, Germany and the US has observed clear evidence of quantum mechanical interference behaviour in collisions between a methane molecule and a gold surface. As well as extending the boundaries of quantum effects further into the classical world, the team say the work has implications for surface chemistry, which is important for many industrial processes.
The effects of interference in light are generally easy to observe. Whenever a beam of light passes through closely-spaced slits or bounces off an etched grating, an alternating pattern of bright and dark intensity modulations appears, corresponding to locations of constructive and destructive interference, respectively. This was the outcome of Thomas Young’s original double-slit experiment, which was carried out in the 1800s and showed that light behaves like a wave.
For molecules and other massive objects, observing interference is trickier. Though quantum mechanics decrees that these also interfere when they scatter off surfaces, and a 1920s version of Young’s double-slit experiment showed that this was true for electrons, the larger the objects are, the more difficult it is to observe interference effects. Indeed, the disappearance of such effects is a sign that the object’s wavefunction has “decohered” – that is, the object has stopped behaving like a wave and started obeying the laws of classical physics.
Similar to the double-slit experiment
In the new work, researchers led by Rainer Beck of the EPFL developed a way to observe interference in complex polyatomic molecules. They did this by using an infrared laser to push methane (CH4) molecules into specific rovibrational states before scattering the molecules off an atomically smooth and chemically inert Au(111) surface. They then detected the molecules’ final states using a second laser and an instrument called a bolometer that measures the tiny temperature change as molecules absorb the laser’s energy.
Using this technique, Beck and colleagues identified a pattern in the quantum states of the methane molecules after they collided with the surface. When two states had different symmetries, the quantum mechanical amplitudes for the different pathways taken during the transition between them cancelled out. In states with the same symmetry, however, the pathways reinforced each other, leading to an intense, clearly visible signal.
The researchers say that this effect is similar to the destructive and constructive interference of the double-slit experiment, but not quite the same. The difference is that interference in the double-slit experiment stems from diffraction, whereas the phenomenon Beck and colleagues observed relates to the rotational and vibrational states of the methane molecules.
A rule to explain the patterns
The researchers had seen hints of such behaviour in experiments a few years ago, when they scattered methane from a nickel surface. “We saw that some rotational quantum states were somewhat weakly populated by the collisions while other states that were superficially very similar (that is, with the same energy and same angular momentum) were more strongly populated,” explains Christopher Reilly, a postdoctoral researcher at EPFL and the lead author of a paper in Science on the work. “When we moved on to collisions with a gold surface, we discovered that these population imbalances were now very pronounced.”
This discovery spurred them to find an explanation. “We concluded that we might be observing a conservation of the reflection parity of the methane molecule’s wavefunction,” Reilly says. “We then set out to test it for molecules prepared in vibrationally excited states and our results confirmed our hypothesis spectacularly.”
Because the team’s technique for detecting quantum states relies on spectroscopy, Reilly says the “intimidating complexity” of the spectrum of quantum states in a medium-sized molecule like methane was a challenge. “While our narrow-bandwidth lasers allowed us to probe the population in individual quantum states, we still needed to know exactly which wavelength we need to tune the laser wavelength to in order to address a given state,” he explains.
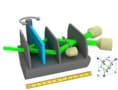
Our world (still) cannot be anything but quantum, say physicists
This, in turn, meant knowing the molecule’s energy levels very precisely, as they were trying to compare populations of states with only marginally different energies. “It is only just in the last couple years that modelling of methane’s spectrum has become accurate enough to permit a reliable assignment of the quantum states involved in a given infrared transition,” Reilly says, adding that the HITEMP project of the HITRAN spectroscopic database was a big help.
Rethinking molecule-surface dynamics
According to Reilly, the team’s results show that classical models cannot fully capture molecule-surface dynamics. “This has implications for our general understanding of chemistry at surfaces, which is where in fact the majority of chemistry relevant to industry (think catalysts) and technology (think semiconductors) occurs,” he says. “The first step of any surface reaction is the adsorption of the reactants onto the surface and this step often requires the overcoming of some energetic barrier. Whether an incoming molecule will adsorb depends not only on the molecule’s total energy but on whether this energy can be effectively channelled into overcoming the barrier.
“Our scattering experiments directly probe these dynamics and show that, to really understand the different fundamental steps of surface chemistry, quantum mechanics is needed,” he tells Physics World.