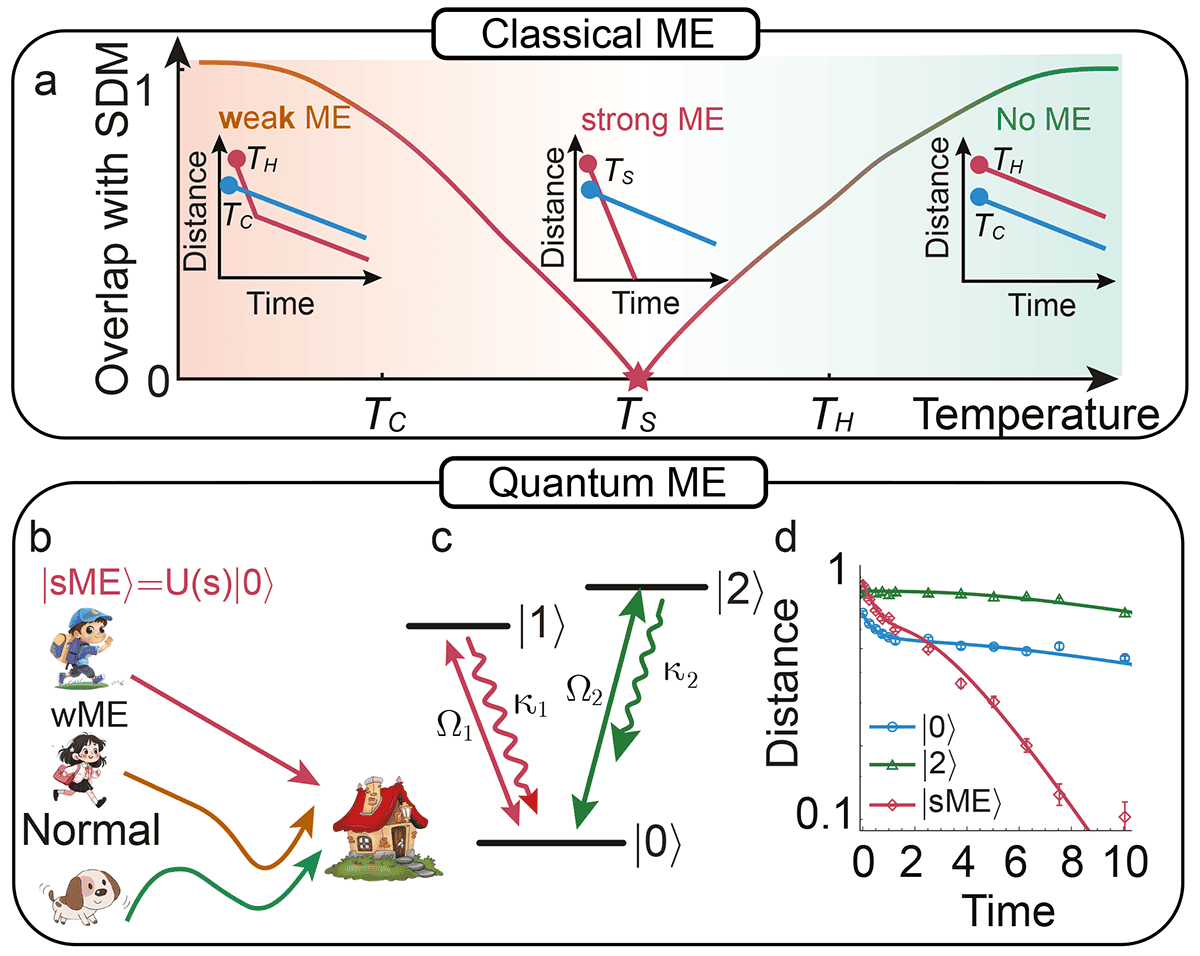
Researchers from China, the UK and Singapore have demonstrated for the first time that choosing the right set of initial conditions can speed up the relaxation process in quantum systems. Their experiments using single trapped ions are a quantum analogue of the classical Mpemba effect, in which hot water can, under certain circumstances, cool faster than cold water. By showing that it is possible to exponentially accelerate the relaxation of a pure state into a stationary state – the hallmark of the so-called strong Mpemba effect – they also provide strategies for designing and analysing open quantum systems such as those used in quantum batteries.
In both the classical and the quantum worlds, the difference between the relaxation process of a system in a strong Mpemba effect (sME) state and any other state is that the decay rate of a sME state is greater than the others. This naturally leads to the conclusion that initial conditions influence the speed at which a system will reach equilibrium. However, the mathematics of the quantum and classical sME are different. While in the classical world an open system is described by the Fokker-Planck equation, with the temperature as the key variable, in the quantum world the Lindblad master equation applies, and the energy of the sME state is what matters.
Paths and overlap
To understand why a quantum system in a particular initial state reaches a steady state faster than any other, we should think about the possible paths that a system can take. One key path is known as the slowest decay mode (SDM), which is the path that takes the system the most time to decay. At the other extreme, the fastest relaxation path is the one taken by a system in the sME initial state. This relaxation path must avoid any overlap with the SDM’s path.
Hui Jing, a physicist at China’s Hunan Normal University who co-led the study, points out that a fundamental characteristic of the quantum sME is that its relaxation path includes the so-called Liouvillian exceptional point (LEP). At this point, an eigenvalue of the dynamical generator, which is the Liovillian superoperator that describes the time evolution of the open quantum system through the Lindblad master equation, changes from real to complex. When the eigenvalue of the SDM is real, the system is successfully prepared in the sME. When the eigenvalue of the SDM acquires an imaginary part, the overlap between the prepared sME state and the SDM is no longer zero. The LEP therefore signals the transition from strong to weak Mpemba effect.
Experimental set-up
To create a pure state that presents zero overlap with the SDM in an open quantum system, Jing and colleagues trapped a 40Ca+ ion and coupled three of its energy levels through laser interactions. The first laser beam, with a wavelength of 729 nm, coupled the ground state with the two excited states with coupling strengths characterized by Rabi frequencies Ω1 and Ω2. A second, circularly polarized laser beam at 854 nm controlled the decay between the first excited state and the ground state.
By tuning the Rabi frequencies, researchers were able to explore different relaxation regimes of the system. When the ratio between the Rabi frequencies was much smaller or bigger than the LEP, they observed the sME and weak ME, respectively. When the ratio equalled the LEP, the transition from sME to weak ME took place.
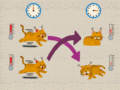
Quantum Mpemba effect hints at faster quantum computers
This work, which is described in Nature Communications, marks the first experimental realization of the quantum strong Mpemba effect. According to Jing, the team’s methods offer an experimental alternative to existing ways of increasing the ion cooling rate or enhancing the efficiency of quantum batteries. Now, the group plans to study how the quantum Mpemba effect behaves at the LEP, since this point could lead to faster decay rates.