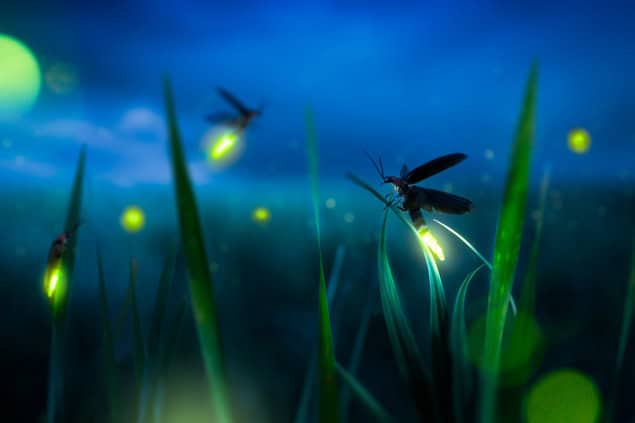
The synchronized flashing of fireflies in summertime evokes feelings of marvel and magic towards nature. How do they do it without a choreographer running the show?
For some physicists, though, these natural fireworks also raise other questions. If the fireflies were quantum, they wonder, would they synchronize their flashing routines faster or slower than their classical counterparts?
Questions of this nature – about how quantum systems synchronize, the energetic costs they pay to do so, and how long it takes them to fall into lockstep – have long bedevilled physicists. Now a team of theorists in the US has begun to come up with answers. Writing in Physical Review Letters, Maxwell Aifer and Sebastian Deffner of the University of Maryland Baltimore County (UMBC), together with Juzar Thingna of the University of Massachusetts, Lowell (UMass Lowell), present a new take on the energetic cost and time required to synchronize quantum systems. Among other findings, they conclude that quantum systems can synchronize in scenarios where such behaviour would be impossible classically.
How quantum springs synchronize
Studies of synchronization go back to the 1600s, when Christiaan Huygens documented that pendulums placed on a table eventually sway in unison. Huygens called this the “sympathy of pendulums”.
This apparent sympathy between systems – chirping crickets, flashing fireflies, the harmonious firing of pacemaker cells in our hearts – turns out to be ubiquitous in nature. And while it may look like magic, it ultimately stems from information exchanged between individual systems via communication pathways (such as the table in the case of Huygens’ pendulums) available in the shared environment.
“At its core, synchronization is about balance of forces,” Thingna says.
To understand how that balance works, imagine you have a bunch of systems moving in circles of different sizes. The radius of the circle corresponds to the amount of energy in that system.
At first, the systems may all be moving at different paces: some faster, others slower. To synchronize, the circles must interact in such a way that gradually, the radius of all the circles and the pace of the systems becomes the same – meaning that bigger circles must “leak” energy and smaller circles gain it.
But synchronization is impressive only when it is resilient and robust. This means that if there are small disturbances – for example, if one of the systems is kicked out of its circle – the disturbed system should return to the radius and pace of the others.
This picture works for classical systems, but synchronization in the quantum regime is more complex. “The challenge lies in translating the classical concept of synchronization to the quantum world where trajectories are ill-defined concepts due to Heisenberg’s uncertainty principle,” explains Christopher Wächtler, a quantum physicist at the University of California, Berkeley, US who was not involved in this work.
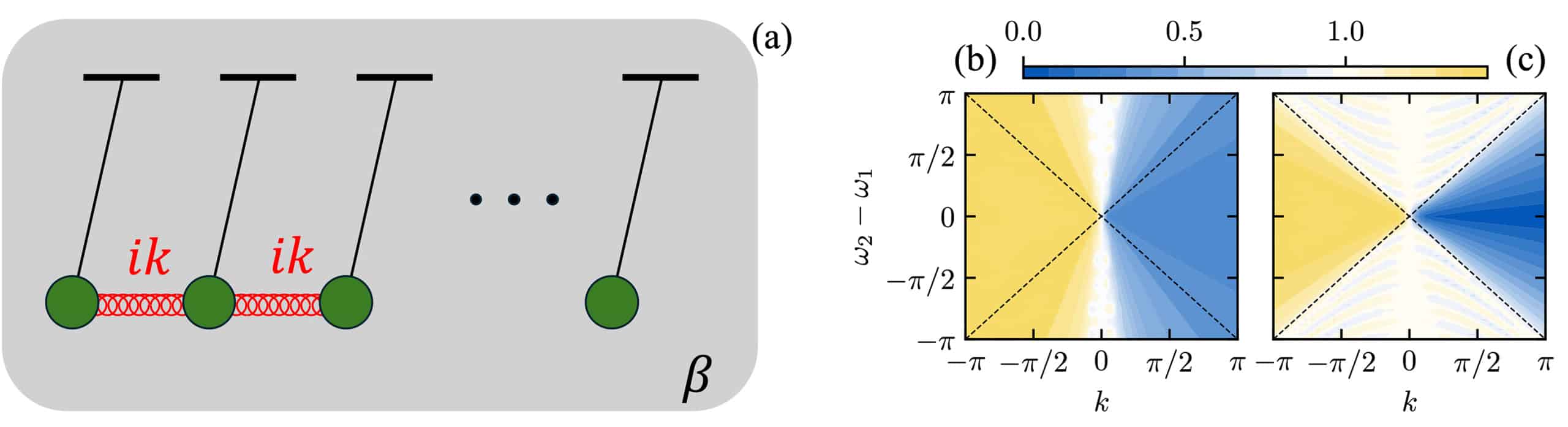
Taking inspiration from an experimental setup, the UMBC-UMass Lowell team created a model based on quantum oscillators or springs (a well-known quantum system) that interact with each other via a biased channel – an anti-Hermitian coupling in which one oscillator is favoured more than the other. This biased channel controls the flow of energy in and out of the individual springs. The oscillators also leak information by “talking” to a common thermal environment at a given temperature.
Thanks to this combination of a common thermal environment and a biased inter-system communication channel, the team was able to balance the information flow (that is, the communication between the oscillators and communication with the environment) and synchronize quantum systems in a way similar to how classical systems are synchronized.
The economics of sympathy
This approach is unusual because quantum synchronization research typically explores the quantum systems in their synchronized state after they have been coupled for a long time. In this case, however, the researchers focus on the time before the steady state has been reached, “which in my opinion is an important question to ask,” says Christoph Bruder, a physicist at the University of Basel, Switzerland who was not involved in the study.
To estimate the time it takes to synchronize, the UMBC-UMass Lowell researchers use quantum speed limits, which are a mathematical way of deriving the maximum time it takes a system to go from an initial state to a desired final state. They find that quantum oscillators synchronize the fastest when the conversations between oscillators do not leak – that is, the strength of the interaction between the oscillators outweighs the interaction with the environment.
The team also used ideas from quantum thermodynamics to identify a lower bound on the energetic cost of synchronization. This bound depends on the biased way in which the oscillators talk to each other.
But there is no free lunch.

The secret of the synchronized pendulums
While synchronizing a small number of quantum systems is energetically more efficient than doing the same for a classical counterpart, the researchers report that this is not scalable. When there are many systems, classical systems are more energy efficient than quantum ones. However, the researchers found that a model system does exhibit quantum synchronization for a wider range of interaction strengths than is the case for classical oscillators, making synchronization in the quantum regime more resilient and robust.
Though the work is still theoretical at this point, Wachtler says that a minimal version of the team’s model could be “effectively implemented in a lab”. The team is keen to explore this further. “For us, this is the first stepping-stone towards this goal of how to make synchronization more practical,” Thingna says.