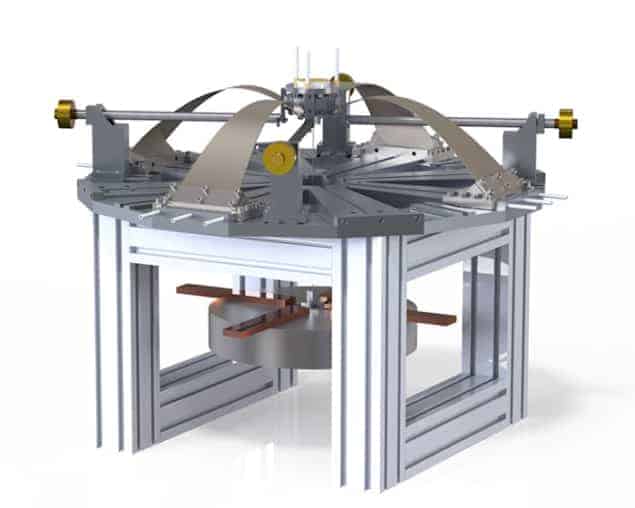
Physicists in Australia have made a high-precision sensor that can measure gravitational and magnetic fields at the same time. The device uses an atom interferometer to track the motion of a Bose–Einstein condensate (BEC) in free fall and the researchers say it could improve the search for iron ore, hydrocarbons, diamonds and other minerals.
Geological exploration often involves making local measurements of the Earth’s gravitational field. In a classical sensor, this involves dropping a mirror a certain distance and using light-based interferometry to measure its height at several points during its fall. However, because this approach involves many moving parts it is not well suited to vibrational, noisy environments.
Quantum sensors, on the other hand, use atom interferometry to measure the free fall of cold atoms. Developed over the past decade and now sold commercially, these devices have surpassed the sensitivity of their classical counterparts. However, like classical sensors, they are at the mercy of ambient noise – and particularly stray magnetic fields. According to Nicholas Robins of the Australian National University (ANU) in Canberra, scientists go to great lengths to shield these devices from stray magnetism in order to minimize noise levels.
Two in one
In the latest work, Robins and colleagues at the ANU have made this magnetic sensitivity a virtue and created a quantum sensor that can measure changes in both accelerations due to gravity and those due to magnetic fields. Not only does this provide accurate gravitational data, they say, but it also yields magnetic data that could be very useful for mineral prospecting.
The new technique involves cooling a sample of rubidium-87 atoms to just a few billionths of a degree above absolute zero in order to turn them into a BEC – a state of matter in which all constituent particles share the same quantum state. The BEC cloud of atoms is then placed in a quantum superposition of three magnetic spin states and allowed to fall under the effect of gravity for several metres. During that time, the height of the cloud is measured three times using an atom interferometer, a device that exploits the wave nature of matter to make extremely precise measurements on atoms.
The advantage of using a BEC, explains Robins’ colleague Kyle Hardman, is that the waves of the constituent atoms remain coherent across the full width of the cloud, which is several millimetres across. This makes it easier to achieve the overlap needed between the two paths of the interferometer to achieve a given sensitivity – thereby allowing the device to work in noisier environments.
Spin superposition
However, Hardman says, the device’s real novelty lies in the spin superposition. Because the three spin states are sensitive to magnetic fields in different directions, the BEC cloud will tend to split into three components as it falls. One state feels magnetic fields pointing upwards, causing it to lag behind during free fall, another is immune to magnetic fields, while the third is subject to downward pointing fields, which boosts it acceleration towards the Earth. The relative size of these three components at the interferometer’s output therefore reveals the magnitude and orientation of local magnetic fields.
To put their device to the test, the Australian group’s researchers created a BEC of two million atoms and ran the interferometer continuously for eight hours, dropping a new BEC every 13 seconds. They looked to see whether they could accurately monitor the varying gravitational acceleration of solid Earth tides – the deformation of the Earth’s crust by the tug of the Moon and the Sun. They also varied the height of their interferometer during the measurement period in order to map variations in the ambient magnetic field. The results of those tests, says Hardman, show their sensor to be “a state-of-the-art gravimeter and state-of-the-art magnetic gradiometer”. Indeed, it is able to measure variations in the acceleration due to Earth’s gravity (g) of one part in a billion.
Elegant extension
Florian Schreck of the University of Amsterdam praises the Australian group for its “interesting and significant” research, which, he says, “elegantly extends the capability of atom interferometers to disentangle the influence of gravity, magnetic field strength and magnetic field gradient”. He believes that a portable version of the new sensor “will be interesting for geology and the prospecting of resources”.
That kind of mineral exploration involves loading an accelerometer on to an aircraft or helicopter and then monitoring the tiny changes in gravitational field that occur as the instrument passes over regions of the Earth. Hardman says that adding magnetic measurements into the mix would yield more detailed crustal maps, but maintains that this can only be done properly if a single device measures both the gravitational and magnetic fields. “If you fly two separate machines it is difficult to merge the information,” he says.
Hardman adds that the team hopes to commercialize the technology, but cautions that they must first overcome a significant technical hurdle: how to reduce the time needed to prepare BECs. Currently standing at around 10 seconds, this limits the rate at which they can make measurements and with it the resolution of their mapping. However, he believes they can do better than the roughly 30 years he says it has taken the mining company Rio Tinto to make classical gravimeters operational. “Cold-atom technology is developing very rapidly,” he says.
The research is described in Physical Review Letters.