
Shuffling around on a carpet to give someone an electric shock might seem like the oldest trick in the book, yet scientists know surprisingly little about why it happens. “I believed – like I think most physicists – that these phenomena were understood by the experts,” says Robert Alicki, a mathematical and theoretical physicist at the University of Gdansk, Poland. “But it was not the case. It was still an open question.”
Thanks to Alicki and his colleague Alejandro Jenkins of the Universidad de Costa Rica, the mystery surrounding triboelectricity (as the “charging by rubbing” effect is known) may be clearing up. According to Alicki and Jenkins, a major barrier to understanding triboelectricity is that physicists tend to view the phenomenon in terms of electrostatic potentials, even though “from a potential effect, you are never going to sustain a current that is going around a circuit,” Jenkins says. “It’s like the problem of perpetual motion.”
Alicki and Jenkins formulated their alternative description by incorporating the concept of pumping into a new, quantum model of a system undergoing triboelectric processes. “Pumping can replenish a potential, but it is not describable by a potential,” Jenkins explains. “It can do something that no potential can do, and that is to drive something around on a closed path.”
Using this pumping-based model, the pair successfully reproduced several experimentally observed characteristics of triboelectricity, such as its dependence on material surface and geometry and the speed of rubbing. In particular, the model accurately predicts that the most electrically negative and electrically positive materials will have symmetrical maximum charge densities when rubbed – something that models based on electric potentials models cannot explain. The new model also predicts a maximum tribovoltage in terms of the sliding velocity of the two surfaces, which Alicki and Jenkins say could be tested using an experimental set-up with sufficient control over a constant sliding velocity.
From lasing bosons to fermions
While Alicki has been working on quantum thermodynamics for decades, Jenkins is a more recent recruit, having started out in high-energy theory. As their paths converged – Jenkins has just begun a fellowship at Gdansk’s Institute for Theory of Quantum Technologies (ICTQT) – they discovered that they shared an interest in systems found in motors and engines that operate away from equilibrium, where energy is irreversibly converted from one form to another. While such systems are the bread and butter of engineers, and Alicki and collaborators started working on them as far back as the late 1970s, Jenkins says that on the whole, they have attracted less attention from theorists than systems at equilibrium, fluctuating around equilibrium or relaxing to equilibrium.
At first, this common interest in out-of-equilibrium systems led Alicki and Jenkins to formulate a mathematical description of “superradiance”, or the enhanced effects of radiation associated with rotating objects. Such effects were first described in 1971 by Yakov Zel’dovich, whose suggestion that superradiance ought to apply to a spinning gravitational mass led to follow-up work by Jacob Bekenstein and Stephen Hawking on the thermodynamics of black holes.
By describing rotating systems in terms of quantum fields, and treating the moving object as a heat bath, Alicki and Jenkins showed how work could be extracted via stimulated emission, similar to a laser’s operation. But while their laser analogy offered a new perspective on such systems, the underlying process, while exotic, was already pretty well-understood. It was only later that they realized that their formulation of a quantum field and two heat baths could lead to something “qualitatively new”: a description for the motion that drives an active current of fermionic electrons from one material to another in the humble triboelectric effect.
“The soul of inanimate objects”
The pair built up their model by defining Hamiltonians with creation and annihilation operators for electron states on the surface of the moving material (where the population inversion takes place) and within the interior of the two rubbed materials (which act as the heat baths). They then defined the pumping of the system in terms of the rate of change of the populations of these electron states. Although the Pauli exclusion principle forbids fermions such as electrons to exhibit superradiance, Alicki and Jenkins were able to show that with the bulk bodies of the two surfaces acting as two heat baths, a motion-induced population inversion of fermions could nevertheless result and sustain a macroscopic current.
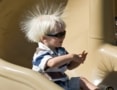
Can flexoelectricity explain the charging by friction conundrum?
While magnetic and triboelectric effects have been known to scientists since antiquity – in the 6th century BCE, the pre-Socratic philosopher Thales of Miletus referred to them as “evidence of a kind of soul” – Jenkins notes that “the interesting point is neither can be described classically”. The need for quantum mechanics to explain the behaviour of permanent magnets was established by Niels Bohr and Hendrika Johanna van Leeuwen over 100 years ago, and Jenkins says that his and Alicki’s latest work shows that the same is true of triboelectricity. Although pumping and work cycles exist in classical thermodynamics, the pair insist that only a quantum treatment can make sense of electrons’ fermionic behaviour as they move between surfaces in the triboelectric effect. In effect, Alicki says, “Quantum mechanics is the soul of inanimate objects.”
Alicki and Jenkins are now considering ways to further investigate dry friction to explore how it relates to the triboelectric effect. They are also interested in understanding details of energy transduction in active devices such as batteries, solar cells and thermoelectric generators, as well as active processes in various applications from astrophysics and cosmology, to fundamental physics.
Full details of the work are reported in Physical Review Letters