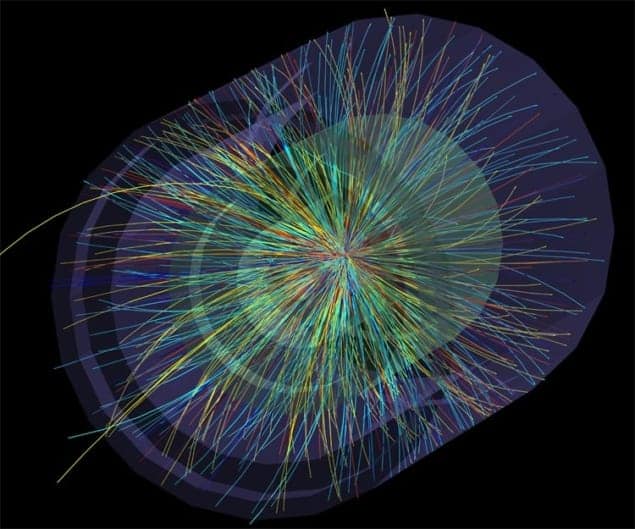
After a successful run of eight months – including recent collisions that could shed light on the primordial universe – the last beam of 2010 was extracted yesterday evening from CERN’s Large Hadron Collider (LHC). Since 7 November the LHC has been colliding lead ions at energies of around 0.5 PeV, 80 times higher than generated by earlier proton collisions. This creates a subatomic blob so hot and dense that nuclear matter dissolves into its constituent quarks and gluons – a state of matter that dominated the universe shortly after the Big Bang.
The search for such a quark–gluon plasma (QGP) first hit the headlines in 2000 when “fixed target” heavy-ion experiments at CERN found evidence for a new state of matter – apparently scooping Brookhaven National Laboratory in the US, where a dedicated QGP machine called the Relativistic Heavy Ion Collider (RHIC) was just starting up. But then in 2005 RHIC announced that its quark–gluon gloop behaved not like a gas, as expected, but like a liquid with zero viscosity. Earlier this year RHIC physicists confirmed that the primary participants in the flow are indeed quarks and gluons, not hadrons.
With the LHC now having created conditions 14 times as energetic as those at RHIC, quark–gluon mania returns to Europe. After a few days of running, the LHC’s dedicated heavy-ion experiment ALICE found evidence for a hot, dense state that still flows like a fluid despite the higher temperatures (arXiv: 1011.3914v1), and revealed a marked increase in the number of particles created in the collisions (arXiv: 1011.3916v2). Taken together, says CERN, these results rule out some theories about how the primordial universe behaved.
Evolution of the infant universe
Theorist Thomas Schaefer of North Carolina State University says that linking the LHC collisions with the evolution of the infant universe is not straightforward, however. “We verified the basic picture (the quark–gluon plasma really exists), we learned very interesting things about it such as its perfect fluidity, but neither of these things directly affects the dynamics of the early universe such as big bang nucleosynthesis,” he told physicsworld.com.
Brookhaven’s Steve Vigdor says the ALICE results certainly suggest liquid behaviour with low viscosity, but he thinks it premature to claim that this “confirms” the near-perfect liquid picture. “The question at this point is what the magnitude of the sheer viscosity of the matter is – how close is it to the conjectured lower quantum limit?” he said. “It’s taken much analysis of RHIC data to start to pin down this question quantitatively; LHC is not there yet.”
The two general purpose detectors at the LHC – ATLAS and CMS – have also brought new perspective on the quark–gluon state. At a seminar held at CERN last Thursday, representatives from ATLAS and CMS reported direct observations of “jet quenching” – when a collimated stream of hadrons created almost instantaneously from the decays of quarks and gluons is swamped as it traverses a dense quark–gluon state. “Jet quenching presumably teaches us about how energetic quarks and gluons interact in the QGP, and should help elucidate the quark–gluon correlations that lead to low-viscosity liquid flow,” says Vigdor.
ATLAS published its result last Monday (arXiv: 1011.6182) and CMS is expected to follow suit once the full heavy-ion dataset has been analysed. At CERN’s heavy-ion seminar last week, ALICE spokesman Jurgen Schukraft stated that the search for the QGP is essentially over, its discovery is well under way, and measuring its properties is just beginning.
QGP in proton collisions?
The LHC has added a further twist in the tale of the QGP. In July, when the machine was perfecting its main job of firing protons at each other, researchers on the CMS detector found that some of the debris from certain collisions containing a large number of particles was correlated – pairs of particles were flying out at angles which suggested they influenced each other at the point of the proton–proton collision. Members of the 3000-strong CMS collaboration claimed in September (arXiv: 1009.4122v1) that they had observed a “potentially new and interesting effect” reminiscent of similar features seen by experiments at RHIC that were interpreted as being due to the presence of hot and dense matter.
“Reminiscent is not a quantifiable scientific measure,” says RHIC physicist Michael Tannenbaum. “In contrast to the great physics that is the discovery of jet quenching at the LHC, which is very strong evidence that a QGP is also produced, claims for the discovery of a new effect in the CMS two-particle correlations are uninformed and inadequately researched.” In a comment about the CMS result (arXiv: 1010.0964v1), Tannenbaum lists several checks that must be made before evidence for a QGP in proton collisions can be claimed, for example concerning features of the QGP observed in gold–gold collisions at RHIC.
Most people are now convinced that a transition from the nuclear matter state to QGP has been seen in relativistic heavy ion reactions Richard Weiner, University of Marburg
Tannenbaum’s co-author, Richard Weiner of the University of Marburg in Germany, says the CMS observation is in line with both RHIC’s findings and previous observations in particle physics made at CERN in the late 1970s, which were interpreted by some as possible evidence for QGP. “Most people are now convinced that a transition from the nuclear matter state to QGP has been seen in relativistic heavy-ion reactions,” he said. “At RHIC this effect has been interpreted in hydrodynamical terms, and the same interpretation applies to proton–proton reactions.” Weiner says that even for many heavy-ion people this is a surprise, yet on the other hand he says many particle physicists have difficulties in accepting the interpretation due in part to the ever-increasing specialization in high-energy physics.
CMS member Pierre Van Mechelen of the University of Antwerp says that the CMS collaboration just reported what it measured, and cautions that this is a new energy domain. “Many models seem to be able to explain the correlations qualitatively, but the real challenge is to reproduce the result seen by CMS in exact numbers,” he said.
Subtle business
Interpreting LHC collisions is a subtle business, though. Protons are only a tiny part quark, while almost all of their mass comes from a sea of fluctuating gluons whose lifetimes at LHC energies are dilated to the point where proton collisions can be viewed as a clash of randomly configured gluonic “hot spots”. Experimentalists have to piece together the underlying physics of quark and gluon interactions, as described by quantum chromodynamics (QCD), from a bucket full of jets and junk produced almost immediately in the mêlée. “The CMS ridge is not predicted by any of the current, widely used QCD Monte Carlo models for proton–proton scattering,” says CMS deputy physics co-ordinator Guenther Dissertori. “For the non-heavy-ion people (the large majority), it was a complete surprise.”
Brookhaven’s Raju Venugopalan, who thinks a framework of high-energy QCD called a colour glass condensate can explain key features of both the CMS result and the ridge events seen in gold–gold collisions at RHIC (arXiv: 1009.5295v2), says that understanding the detailed structure of the CMS ridge provides a unique snapshot into the microscopic structure of visible matter. “Clearly, this novel phenomenon has triggered a rash of papers and will continue to do so, but few people have so far considered the systematics of the effect.”
Whether the CMS two-particle correlations are due to a colour glass condensate, a quark–gluon plasma, a rotating (arXiv: 1009.5229v3) or an exploding (arXiv: 1009.4635v1) deconfined quark–gluon state, or perhaps gluodynamic quantum entanglement (arXiv: 1010.4463v1), physicists ultimately have to be able to account for it if they are to disentangle signal from background when searching for new particles. The LHC beam may be down until February, when protons will be reinstated, but the task of interpreting its first year of data is far from over.