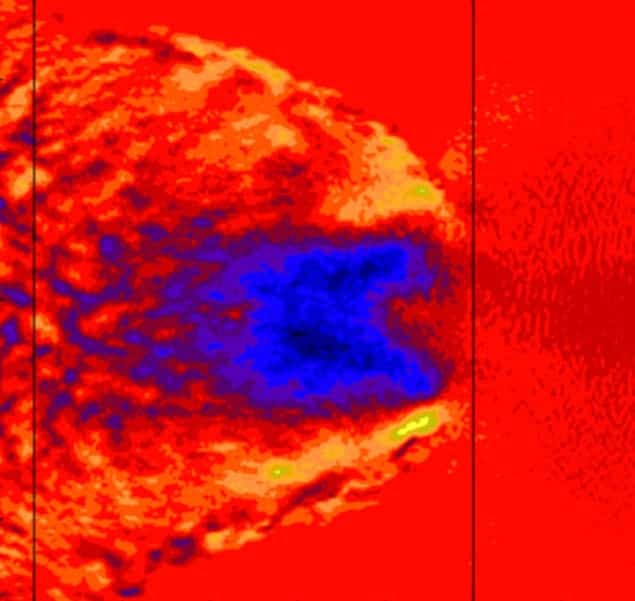
Huge magnetic fields can be created by firing intense, circularly polarized laser pulses at a target, according to calculations by physicists in Italy, Germany and Russia. Their research suggests that the mysterious phenomenon of “radiation friction” plays a crucial role in generating the field. Measuring such magnetic fields could offer physicists a new way of studying this poorly understood effect, which is believed to play a crucial role in the physics underlying astrophysical objects such as gamma-ray flares.
Physicists have long known that a charged particle, such as an electron, emits electromagnetic radiation when it is accelerated – a phenomenon that makes synchrotron radiation facilities and free-electron lasers possible. As energy is conserved, the charged particle loses energy as it radiates and this can be thought of as radiation friction (RF). As well as playing a key role in gamma-ray flares, RF also affects how energy is dissipated in laser-plasma experiments whereby laser pulses are fired at a solid target to create a plasma in which electrons are accelerated to high energies.
Poor models
While experimental studies of RF have provided some information about the phenomenon, physicists do not have good models for calculating its effects. This is why Andrea Macchi at the University of Pisa, Tatyana Liseykina of the University of Rostock and Sergey Popruzhenko at the Moscow Engineering Physics Institute have come up with a new proposal for an experiment that could help to nail down RF.
Calculations and simulations done by the trio show that intense light pulses produced by next-generation, multi-petawatt or exawatt lasers could create short-lived multi-gigagauss magnetic fields in a plasma. Such fields are billions of times stronger than the Earth’s magnetic field and are created when charged particles in the plasma are accelerated into circular orbits. Crucially, the trio’s work shows that RF is the dominant mechanism whereby the charged particles absorb angular momentum from the pulse. Measuring the magnetic fields would therefore provide a direct probe of RF.
Persistent field
This magnetic field is expected to persist for much longer than the duration of the laser pulse that created it, meaning that the field could be detected using optical polarimetry. This involves sending a much weaker pulse of polarized laser light through the plasma and measuring the rotation of the polarization of the transmitted light, which is caused by the presence of the magnetic field. Macchi told physicsworld.com that “this technique has been previously used successfully with sub-picosecond resolution in laser-produced plasmas.”
Although there have been other proposals for measuring RF, Macchi says that in his team’s case, “the RF signature is robust because without RF there would be no strong magnetic field, so that discriminating RF effects may be less prone to experimental uncertainties.”
Macchi says that a better understanding of RF would be useful to astrophysicists who are trying to recreate in the lab the extreme conditions that occur near astronomical objects such as pulsars. Vast amounts of radiation are created in such regions, which comprise strongly magnetized and radiation-dominated plasmas. A better understanding of the behaviour of charged particles in such regions could also shed further light on how extremely high-energy cosmic rays are created in these environments.
Next-generation limit
Astrophysicists will probably have to wait for the next generation of pulsed lasers to be built, however. “Our calculations suggest that at least a power of several petawatts is needed, which is at the limit of the expected performance for forthcoming lasers such as APOLLON, ELI or XCELS,” says Macchi. However, he is not yet ruling out experiments at existing facilities: “We have not explored yet all the possible range of laser and plasma parameters, thus it might be that more accessible conditions are found.”
The research is described in the New Journal of Physics.