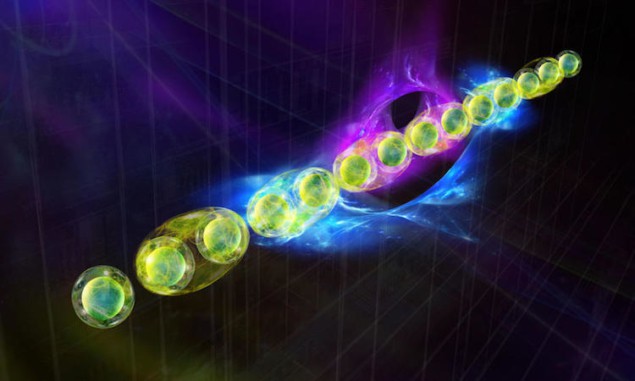
Topological materials – materials that have surface properties very different to those found in their bulk – are currently revolutionizing condensed-matter physics thanks to their unique characteristics. Researchers in Austria, France, the US and Germany, have now put forward a new technique to identify and characterize the global invariants that mathematically describe these materials in various experimental platforms in the laboratory. The work could advance our understanding of these structures, which might be used in next-generation energy-efficient electronics and quantum-computing applications.
Topology – sometimes called “rubber sheet geometry” – is a branch of mathematics in which two objects are assumed to be equivalent if they can be continuously deformed into one another by bending, twisting, stretching or shrinking (but not tearing or cutting). In this framework, a circle is topologically equivalent to an ellipse, for example, and a doughnut to a coffee mug. In both cases, the objects can be deformed into the other by stretching.
Topological materials show similar geometries on the molecular scale, which gives rise to several unusual mechanical and electrical properties. Topological insulators, for example, do not carry electrical currents in their bulk, but current does flow along their surfaces through special “edge” states. Crucially, the electrons in these states can only travel in one direction, and they also steer around imperfections or defects on the surface without backscattering. Since backscattering is the main energy-dissipating process in electronic devices, these “topologically protected states”, as they are known, might be useful ingredients in next-generation energy-efficient devices.
Another benefit is that in topological materials, a surface electron with a certain momentum cannot scatter into a state with opposite momentum because to do so it would have to flip its spin. Topologically protected states might thus also be ideal for quantum-computing applications, in which defects usually destroy quantum information (the spin state) carried by electrons.
Topological invariants
While characterizing these topologically protected states is important for classifying the different topological phases that can be realized for potential applications, doing so in laboratory experiments is difficult. This is because the mathematical invariants that describe them are considered as global, overall, quantities and thus cannot be probed on small, local scales.
A team of physicists led by Peter Zoller from the Centre for Quantum Physics at the University of Innsbruck and the Institute for Quantum Optics and Quantum Information at the Austrian Academy of Sciences and Benoit Vermersch from the University of Grenoble-Alpes in France have now put forward a new measurement technique that overcomes this problem.
“The topological invariants in these states of matter are very complex functions of their quantum states, which makes a direct measurement in an experiment an impossible task,” explains Vermersch. “What we propose instead is to extract ‘many-body’ topological invariants (MBTIs) from a data set, which we obtain from several measurements.”
Random operations
The experimental recipe consists of subjecting a quantum state to a number of different random operations and studying how it reacts, Vermersch says. “By then using random matrix theory, we have proven that we can estimate MBTIs from this data set obtained from such randomized measurements.”
The specific feature of this technique is that although the topological invariants are highly complex, non-local correlation functions, they can still be extracted from statistical correlations of randomized measurements, he adds. Such random measurements are possible in synthetic quantum matter (or “quantum simulators”) made from tried and trusted experimental platforms such as cold atoms, trapped ions and superconducting quantum bits, to name but three. “Our protocol for measuring the topological invariants can therefore be directly studied in these existing systems in the laboratory,” says Vermersch.

Ubiquity of topological materials revealed in catalogues containing thousands of substances
Spurred on by their preliminary results, which they report in Science Advances, the researchers say that they would like to generalize their toolbox to be able to classify different types of topological phases beyond the ones studied in the present work. “Such phases include exotic ones, like those with ‘intrinsic topological order,’” Vermersch tells Physics World. “Doing this will of course be a great technological challenge.”
Vermersch and Zoller worked with colleagues Andreas Elben and Jinlong Yu to develop their measurement technique. They also collaborated closely with Guanyu Zhu and Mohammad Hafezi from the Joint Quantum Institute in Maryland and Frank Pollmann from the Technical University of Munich.