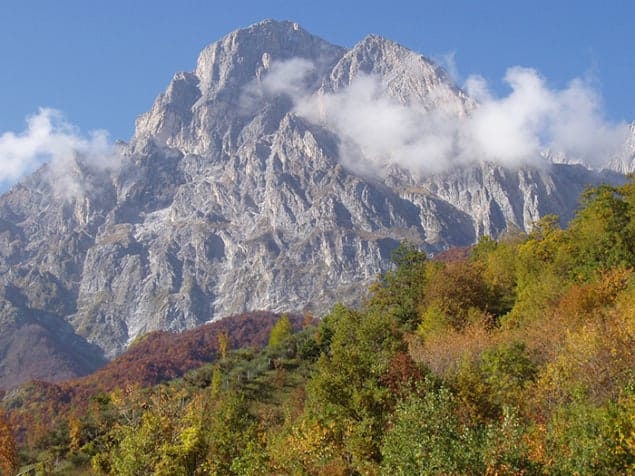
A laser gyroscope located deep beneath the Gran Sasso mountain in central Italy has made the first deep-underground measurements of the rotational motion that passing seismic waves generate in the Earth’s crust. The ability to make such measurements could boost our understanding of the strain that rocks undergo before an earthquake takes place, say the scientists who carried out the research.
Earthquakes release large amounts of pent-up energy in the form of seismic waves, which propagate in all directions from the quake’s epicentre. When those waves reach the Earth’s surface they can cause the ground to move along one or more orthogonal axes – up and down, back and forth, and side to side. But seismic waves can also generate much smaller rotational motions, in which the ground rotates around one or more of the three axes.
According to Gilberto Saccorotti of Italy’s National Institute for Geophysics and Volcanology (INGV), rotational motion is important to measure for a number of reasons. For one thing, seismologists can determine the speed of a seismic wave – and so better understand the kind of rock it propagates through – by comparing the magnitudes of the rotational and translational motions that it generates. In addition, better measurements of ground rotation during strong earthquakes would allow for more robust building regulations. “Circular motion, like horizontal motion, can be very dangerous,” he says. “Structures haven’t been designed with that in mind but are instead meant to resist vertical forces, i.e. their own weight.”
Circular motion, like horizontal motion, can be very dangerous
Gilberto Saccorotti, National Institute for Geophysics and Volcanology
Seismometers are not naturally suited to measuring this circular motion because they are based on pendulums or masses-on-a-spring, which respond in the same basic way, whether the devices’ housing moves up and down or is tilted. While arrays of seismometers can be used to measure circular motion, the relativity weak nature of these events means that only the strongest signals can be detected in this way.
Frequency shift
Ring-laser gyroscopes, on the other hand, are designed specifically to measure rotational motion. These devices record the very tiny differences in frequency between two laser beams sent in opposites directions around an optical circuit that is fixed rigidly to the ground. The frequency offset reflects the rate at which the ground rotates. Ring lasers in Germany, New Zealand and the US have been detecting earthquakes’ rotational ground movements for about the last two decades, but the fact that these instruments are located at or just below ground level exposes them to disturbances – be they of natural or human origin – that originate close to the Earth’s surface.
In the latest work, Saccorotti and colleagues at the INGV and Italy’s National Institute for Nuclear Physics (INFN) used a ring laser called GINGERino, consisting of four 3.6 m-long sides mounted on a block of granite. Housed 1400 m underground at the Gran Sasso National Laboratory, the device is largely shielded from the tiny variations in air pressure that can trouble ring lasers at shallower depths. It is the forerunner of an experiment called Gyroscopes in General Relativity (GINGER), which will use at least three large ring lasers arranged at right angles to one another to try and measure the very subtle “frame-dragging” effect predicted by Einstein’s general theory of relativity.
Using GINGERino, the INFN-INGV group was able to record a magnitude-seven earthquake that occurred under the Atlantic Ocean during a week of data-taking in June 2015. The researchers say that although their data exhibit a poor signal-to-noise-ratio, they were still able to detect rotational motion generated by the earthquake’s seismic waves in the rock surrounding the lab.
Potential earthquake precursors
According to Saccorotti, the result shows the feasibility of installing a long-term experiment in the Gran Sasso lab – be it GINGER or a single, larger ring laser. Such a device would systematically record rotational ground motions over a two- to four-year period. This would allow the detailed study of the elastic deformation of rock caused by the gradual build-up of energy across a geological fault ahead of an earthquake. “That deformation can include rotational motion, so having a very sensitive device in a low-noise environment opens up interesting possibilities for studying a potential earthquake precursor”, he says, pointing out that Gran Sasso is in one of the most seismically active regions of Italy.
Ulrich Schreiber of the Technical University of Munich, who collaborates with the Italian group, points out that the field of “rotational seismology” is now quite well established, thanks to the availability of improved ring-laser gyroscopes. But he nevertheless praises the latest work. “GINGERino is a prototype instrument that has still to mature a fair bit before reaching its full potential,” he says. “But being able to observe rotational motion from remote earthquakes in a deep-underground laboratory is an important step forward.”
The research is reported on the arXiv preprint server.