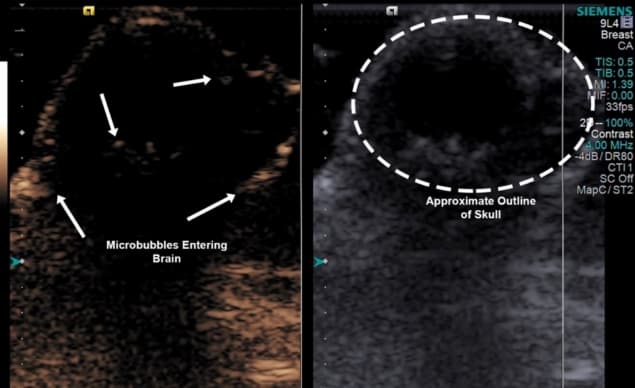
When ruptured by ultrasound, oxygen-filled microbubbles increase the partial pressure of oxygen in malignant tumours, helping to reduce hypoxia. The technique could enhance tumour response to radiotherapy by reducing the radioresistance of hypoxic cancer cells.
Researchers at Thomas Jefferson University have demonstrated that ultrasound-induced microbubble rupture, followed immediately by radiotherapy, significantly impeded the growth of breast cancer brain metastases in mice and extended survival compared with radiotherapy alone. This pilot study establishes the feasibility of further development of the technique for potential clinical use (J. Ultrasound Med. 10.1002/jum.15031).
Led by John Eisenbrey, the research team analysed the outcomes of 15 nude mice assigned to one of five treatment groups. All mice had received injections of brain-seeking breast cancer cells into the right hemispheres of their brains and developed tumours.
A control group received no treatment whatsoever. A second group received 10 Gy of low-linear energy transfer radiation to their tumours, under cone-beam CT guidance. Three groups received microbubble injections before irradiation at 10 Gy: two were injected with oxygen-carrying microbubbles, one of which underwent ultrasound-triggered microbubble rupture immediately before radiotherapy, while the final group was injected with nitrogen-carrying microbubbles, followed by ultrasound-triggered microbubble rupture.
In comparison, tumours treated with oxygen-carrying microbubbles that were not ruptured before radiotherapy exhibited a mean increase of 383% (±226%) in volume. This growth was similar to that of the mice who only received radiotherapy, who showed a mean increase of 337% (±214%).
Interestingly, mice who received ultrasound-ruptured nitrogen-infused microbubbles prior to irradiation also fared better, with only an 80% increase in tumour volume. However, their survival outcomes were poorer than those with oxygen-infused ultrasound-ruptured microbubbles: 3.1 weeks compared with 4.0 weeks, respectively.
The control group survived a median of 2.9 weeks following injection of breast cancer cells. The groups that received radiation only and oxygen-infused microbubble injection followed by radiation had similar median survival outcomes, at 3.9 and 3.7 weeks respectively.
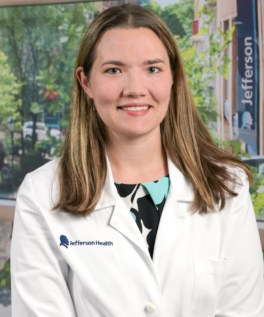
While this pilot study demonstrates the potential positive impact of ultrasound-ruptured oxygen-infused microbubbles, the challenge of tissue oxygenation remains. Lead author Lauren Delaney tells Physics World that the team’s next challenges are to identify methods to deliver more oxygen to the tissues, so that cells can be better sensitized to radiation, and to increase the time during which the cells and tissues are oxygenated. This could enable patients to benefit from increased radiosensitivity during the time span of a radiotherapy treatment.
“One approach that we are considering is the inclusion of tumour mitochondrial respiration inhibitors in the microbubble shell, which will help sensitize the cells to radiation therapy while also interfering with aerobic respiration in the target cells,” says Delaney. “We hope this will prolong the effect of microbubble oxygen delivery long enough to be clinically viable.”
“We could also look into modifying the microbubble fabrication process to produce microbubbles with larger diameters of up to 8 μm,” she added. “And we could investigate augmenting radiotherapy applications where the radiation is already present, such as brachytherapy.” The team is currently investigating the feasibility of each of these approaches.
Delaney says that in future in vivo work, the researchers hope to use high-intensity focused ultrasound, both to visualize microbubbles within brain tissue and to ensure effective microbubble rupture for tissue oxygenation. Meanwhile, they are currently investigating use of an immunocompetent tumour model to overcome tissue hypoxia during radiotherapy. “Research using syngeneic tumour models can help better replicate the tumour microenvironments and immune responses in human patients,” she explains.
Looking ahead to clinical applications of this technique, Delaney emphasizes that the main issue to overcome is the duration of tissue oxygenation, so that patients can retain the benefit of increased radiation sensitivity in the interim time between receiving microbubbles, being scanned with ultrasound to rupture the microbubbles, and then receiving radiation therapy.
“We also need to scale up the production of the microbubbles with a more consistent and mechanized approach, so that we can produce sterile uniform populations of oxygen-loaded microbubbles,” she adds.
After this, preclinical trials could begin, followed by formal clinical trials with cancer patients.