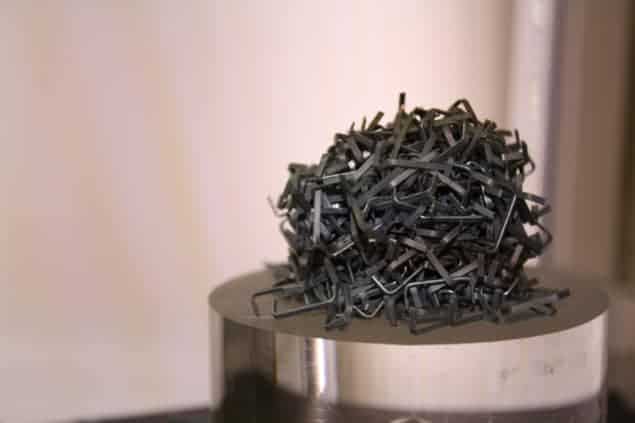
All experimental physicists know that some tasks can be tedious and painstaking, but Nick Gravish from the Georgia Institute of Technology has just taken this to a new level. In a series of experiments to find out more about the behaviour of U-shaped objects, Gravish and colleagues spent about 10 man-hours carefully trimming the arms of thousands of steel staples to see how their shape affects how the staples cling together.
To their surprise, the researchers found that there is an optimum arm length, above and below which the staples are less likely to stick together when shaken. As well as helping scientists to understand materials that are made of U-shaped components, the research could also provide insight into how biological systems as diverse as fire-ant rafts and eagle nests are formed.
Humble but complex
Physicists have a long history of studying the behaviour of granular materials, for example to find out why sand piles collapse or why grains jam when passing through an industrial “hopper”. Some granular materials – including some colloids – can even become entangled with each other and so behave very differently from particles with simple shapes, such as sand grains.
Humble staples are an ideal way of gaining a better understanding of how this entanglement occurs because they are a very simple example of an object that can mechanically grab hold of its neighbours. Carried out by Gravish, Dan Goldman and David Hu at Georgia Tech along with Scott Franklin at the Rochester Institute of Technology, each experiment began with a clump of staples all with the same arm length. The researchers placed the staples in a cylindrical mould that is on top of a vibrating plate. After vibrating the column of staples for about 20 s to ensure that it is in a tightly packed state, the team found that the density of the column was greatest for staples with no arms – essentially rods – and that the density dropped off for staples with longer arms.
The researchers then removed the cylinder before shaking the free-standing column again and monitoring it with a digital camera. As the column collapsed, they measured its height as a function of time, from which they derived the average energy required to disentangle one staple from another.
Long arms
As expected, this “energy barrier” rises as the arm length increases because a staple with a longer arm takes more energy to disentangle. What was surprising, however, was that the energy barrier did not keep on rising, but rather peaked in staples where the arms are about 40% of the width of the staple. For longer arms, the energy appeared to drop off.
When Gravish and colleagues carried out computer simulations of how the staples pack and collapse, they found that another factor was at work. It turns out that in the case of staples with arms longer than about 40% of their width, these objects do not pack as tightly as their shorter counterparts. The result is that there is less entanglement in the column to begin with and so it takes less energy to shake it apart.
While the team’s work should provide clues to the behaviour of materials made of entangled inanimate objects such as some colloids, Gravish and Goldman say that it cannot fully explain how living organisms such as fire ants hook themselves together into structures such as floating mats – which are made to save an entire ant colony in a flood. Instead, they believe that the work will allow biologists to differentiate between purely mechanical effects and behavioural effects that define how such structures are made.
The work is described in Physical Review Letters.