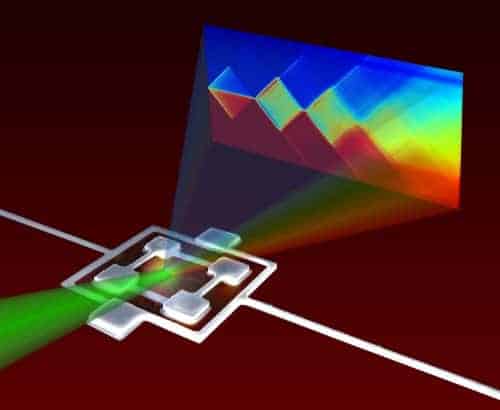
Spectroscopy has come a long way since the early 19th century when the German physicist Josef Fraunhofer first saw dark lines in the spectrum of light coming from the Sun, which give clues to its chemical composition.
The technique is now commonplace in research labs and usually involves shining a beam of light on a sample and watching how it absorbs light as the frequency of the radiation is swept through a range of values. An atom, for example, absorbs radiation at a specific set of frequencies that correspond to gaps between the energy levels of its electrons.
Physicists in the US have now turned the idea of atomic spectroscopy on its head by inventing a new technique that works by shining radiation of a fixed frequency on a sample, while sweeping the amplitude of the radiation up and down.
They say the new method works particularly well on tiny pieces of solid material called “artificial atoms” and that it could someday help researchers build and control quantum computers based on such devices (Nature 455 51).
Tricky microwaves
One problem with conventional spectroscopy is that it can be tricky to carry out with microwaves and millimetre waves (at frequencies of 10–300 GHz). Put simply, it is hard to disentangle the true spectrum of a sample from unrelated frequency-dependent effects in the spectrometer.
This has been bad news for researchers studying artificial atoms, which exhibit properties of ordinary atoms, including discrete energy levels. Such atoms could potentially be used to store and process data. The snag in using artificial atoms as putative quantum-information systems is that the gaps between the levels tend to be in the tricky millimetre and microwave region.
The new technique — devised by William Oliver and colleagues at the Massachusetts Institute of Technology (MIT) in the US — shows great promise for studying, and possibly even controlling, artificial atoms. The team’s artificial atom is a “superconducting qubit”, which is a tiny loop of niobium about 16 µm across that is cooled to about 20 mK. A superconducting current flows around the loop and its energy levels are quantized much like the energy levels of electrons orbiting an atomic nucleus.
Shifting energy levels
The team exposed their qubit to 0.16 GHz radio waves and swept the amplitude of the radiation. As the amplitude increases, the energy levels (or quantum states) of the artificial atom are shifted in energy. This was detected by measuring tiny changes in the superconducting current – or more precisely changes in the magnetic flux through the loop.
Some states are shifted up in energy and some down – and eventually two states can meet. But instead of crossing each other, quantum mechanics requires that an energy gap occurs where the two states intersect. At this point, the qubit can remain in that state, or jump to the other state.
However, in the weird world of quantum mechanics, the qubit is actually in a superposition of both states at the same time. So if the amplitude of the applied radiation is swept up and down in the region of a crossing, the superposition state “collides” with itself, causing an array of interference patterns to appear in a plot of flux versus amplitude.
These interference patterns are contained within a series of “diamonds”. According to Oliver, the energy levels of the artificial atom can be deduced from the position of the diamond vertices along with information extracted from the interference patterns.
A rougher sort of quantum control
Oliver told physicsworld.com that amplitude spectroscopy could lead to new ways of controlling qubits. Today, most quantum-control schemes are adiabatic, using small-amplitude pulses to gently nudge a qubit from one state to another without destroying its quantum nature. However, the MIT researchers found that a brief but large-amplitude pulse at a fixed frequency could be used to switch their qubit, suggesting that quantum control could be achieved using “non-adiabatic” schemes.
The team are now looking into such “non-adiabatic” methods of quantum control.