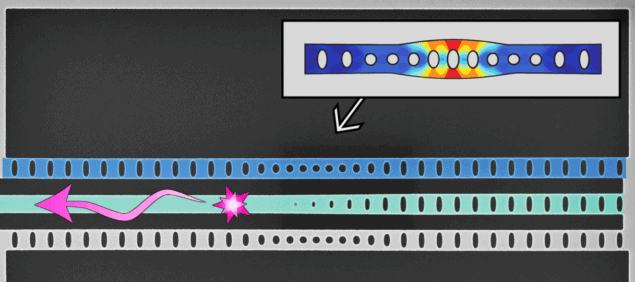
Quantum entanglement in a macroscopic mechanical system has been demonstrated by physicists in Austria and the Netherlands, who confirmed that their experiment passes the “Bell test” for entanglement. The system was created by Simon Gröblacher and colleges at Delft University of Technology and the University of Vienna and could lead to the development of new quantum-information technologies such as memory nodes.
Entanglement is a curious consequence of quantum mechanics that allows two particles to be connected in a way that cannot be described by classical physics. It is normally observed as correlations between measurements made on two particles (such as their polarizations). In 1964 the Northern Irish physicist John Bell described his famous test of whether such correlations are stronger than those allowed by classical physics – as defined by a violation of what is now called Bell’s inequality.
It turns out that entanglement can also occur in systems that are much larger than fundamental particles. Earlier in 2018, Gröblacher’s team showed that it is possible to entangle two identical 10 micron-long pieces of silicon – each containing around 10 billion atoms – that were clamped at each end and functioned as mechanical resonators.
Now, writing in Physical Review Letters, the team says it has used a similar system to perform “the first Bell test using correlations between light and microfabricated mechanical resonators, which constitute massive macroscopic objects”.
Optical interferometer
The test involved putting the resonators into the two separate arms (paths) of an optical interferometer so that the resonators were separated by 20 cm. The interferometer is created using two optical beam splitters – one that splits a beam of light into the two arms and another that combines the two beams before splitting it again and sending two beams to two different photon detectors.
The resonators are cooled to well below 1 K and the experiment begins with a blue laser pulse being fired into the interferometer, which creates vibrations in one of the resonators. This vibrating resonator then emits a photon, which is detected by one of the detectors as it emerges from the interferometer – thereby confirming the excitation. Because the resonators are identical, there is no way of knowing which resonator emitted the photon and this puts the two resonators into a state of entanglement.
Correlated pairs
Then, a second pulse of red light is fired into the interferometer, which causes the vibrating resonator to emit a second photon, which is also picked-up by one of the two photon detectors. Entanglement of the resonators is confirmed by measuring the correlations between which detectors measured the photon pairs – for example, the number of times that a measurement of the first photon in detector-1 was followed by the second photon in detector-2.
Using a phase-shifter in one arm of the interferometer, the team could adjust the phases of the blue and red pulses as they passed through the different arms. By measuring the correlations as a function these phases, they were able to confirm that Bell’s inequality was violated by more than 4σ.
The lifetimes of the excitations in the resonators are currently several microseconds. However, if this could be increased significantly, the system could be used as a memory node in a quantum computer. Gröblacher and colleagues now hope to demonstrate entanglement on even larger scales, and with more complex quantum states of optomechanical resonators.
The experiment is described in Physical Review Letters.